Review Article | Open Access
Targeting CTLA-4 in Cancer: Biological Insights with a Focus on Renal Cell Carcinoma
Juan Wu1, Ya-fei Ren2, Jun Xie1, Dong-sheng Li1
1Department of Rheumatology and Immunology, Jiangxi Province Ganzhou City People's Hospital, Ganzhou City, Jiangxi Province, China.
2Department of Rheumatology and Immunology, Guilin Medical University Affiliated Hospital, Guilin City, Guangxi Zhuang Autonomous Region, China.
Correspondence: Dong-sheng Li (Department of Rheumatology and Immunology, Jiangxi Province Ganzhou City People's Hospital, 17 Hongqi Avenue, Ganzhou 341000, Jiangxi Province, China; Email: lidongsheng2456@sina.com ).
Annals of Urologic Oncology 2022; 5(2): 68-74. https://doi.org/10.32948/auo.2022.12.15
Received: 04 Dec 2022 | Accepted: 14 Dec 2022 | Published online: 21 Dec 2022
Key words immune checkpoint inhibitors, renal cell carcinoma, anti-CTLA-4, biological insights
The ability to evade immune surveillance and programmed cell death is a major mechanism for evasion of cancer [8]. Various tumors may express biomarkers that prevent the host from generating an immune response [9]. In fact, this is essential for that host's regulation of cell proliferation to prevent damage to the nuclear content. It is well known that cell division halts after sustained cell damage to allow repair. When repair is not possible, cell death is induced to prevent the development of defective cell. The rare cases of spontaneous response of RCC provide information that the immune system may be able to suppress RCC through antitumor immunity [10]. Immunotherapy is an increasingly popular and researched treatment that uses the body's own immune system to fight metastatic cancer [9]. Immune checkpoint inhibitors (ICI) upregulate the immune response by blocking programmed cell death protein 1 (PD-1) receptors, ligands of PD-1, or cytotoxic T lymphocyte-associated protein 4 T cells, leading to a new era of immunotherapy [11]. CTLA-4 and PD-1 are inhibitory receptors with molecular significance. A large number of these agents have been developed, studied, and are currently marketed as effective therapies for the treatment of mRCC and many other malignancies. This review describes CTLA-4 in detail.
T cell activation is a complex process involving multiple stimulatory and inhibitory receptors. The initial step requires antigen-specific T cells to recognize MHC peptides, but also requires costimulatory signals from the interaction between membrane proteins on antigen presenting cells, including B7 family members CD 80 (also known as B7-1) and CD 86 (also known as B7-2), and CD28 on T cells [14-16]. A variety of signals influence the activation status of T cells, but cytotoxic T lymphocyte antigen 4 (CTLA-4), programmed cell death 1 (PD-1), and programmed cell death 1 ligand 1 (PD-L1) are the only immune checkpoints that currently have routine clinical application in patients with RCC [17, 18]. In addition, the biological and clinical significance of several other B7 family members is only now being elucidated [17].
The fact that CTLA-4 acts on the cell surface suggests a strategy to enhance T cell immunity by using CTLA-4 inhibitory antibodies. Allison et al. has demonstrated the negative effects of CTLA-4 and established the antagonistic effects of CTLA-4 and CD28 on T cell stimulation. The study showed in detail that binding of CTLA-4 to B7 ligand abolished IL-2 secretion by T cells and T cell proliferation following TCR activation. Blocking CTLA-4 with anti-CTLA-4 antibodies leads to rejection of pre-established tumors, and mice lacking the Ctla4 gene (Ctla4-/-mice) develop severe lymphoproliferative and lethal autoimmune phenotypes [27-29]. Further studies have shown that CTLA-4 is involved in activating an intrinsic signaling cascade in T cells. It has been reported [30-34] that CTLA-4 activation inhibits IL-2 production and T cell proliferation and induces cell cycle arrest through interaction with pathways regulating cell survival and proliferation, including PI3K, NF-κB, and MAPK pathways. In addition, the interaction of anti-CTLA-4 monoclonal antibodies with CTLA-4 may activate antibody-dependent cell-mediated cytotoxicity (ADCC) or complement-mediated lysis. We found that complement activation was lower in patients receiving ipilimumab (IgG1 antibody) than in patients receiving tremelimumab (IgG2 antibody) [35, 36]. Ipilimumab isotype IgG1 has a high affinity for FcγRIIIa, the Fc receptor mediating ADCC. More interestingly, patients with polymorphisms in this receptor were more responsive to ipilimumab [37].
Multiple signals, both stimulatory and inhibitory, modulate the activation of T cells by tumour cells or antigen-presenting cells. Tumour peptides are presented by major histocompatibility complex (MHC) glycoproteins and recognized by antigen-specific T cells. CTLA-4 acts as a negative regulator of T cell activation by binding to B7 ligands CD80 and CD86 expressed on antigen-presenting cells, thereby preventing the co-stimulatory interaction between CD28 and the B7 ligands. Ipilimumab binds to CTLA-4 and blocks the inhibitory signaling of the CTLA-4: B7interaction. PD-1 acts as a negative regulator of T cell activity predominantly by binding to PD-L1 on either tumor cells or antigen-presenting cells, leading to downstream signaling that inhibits anti-tumor T cell responses.
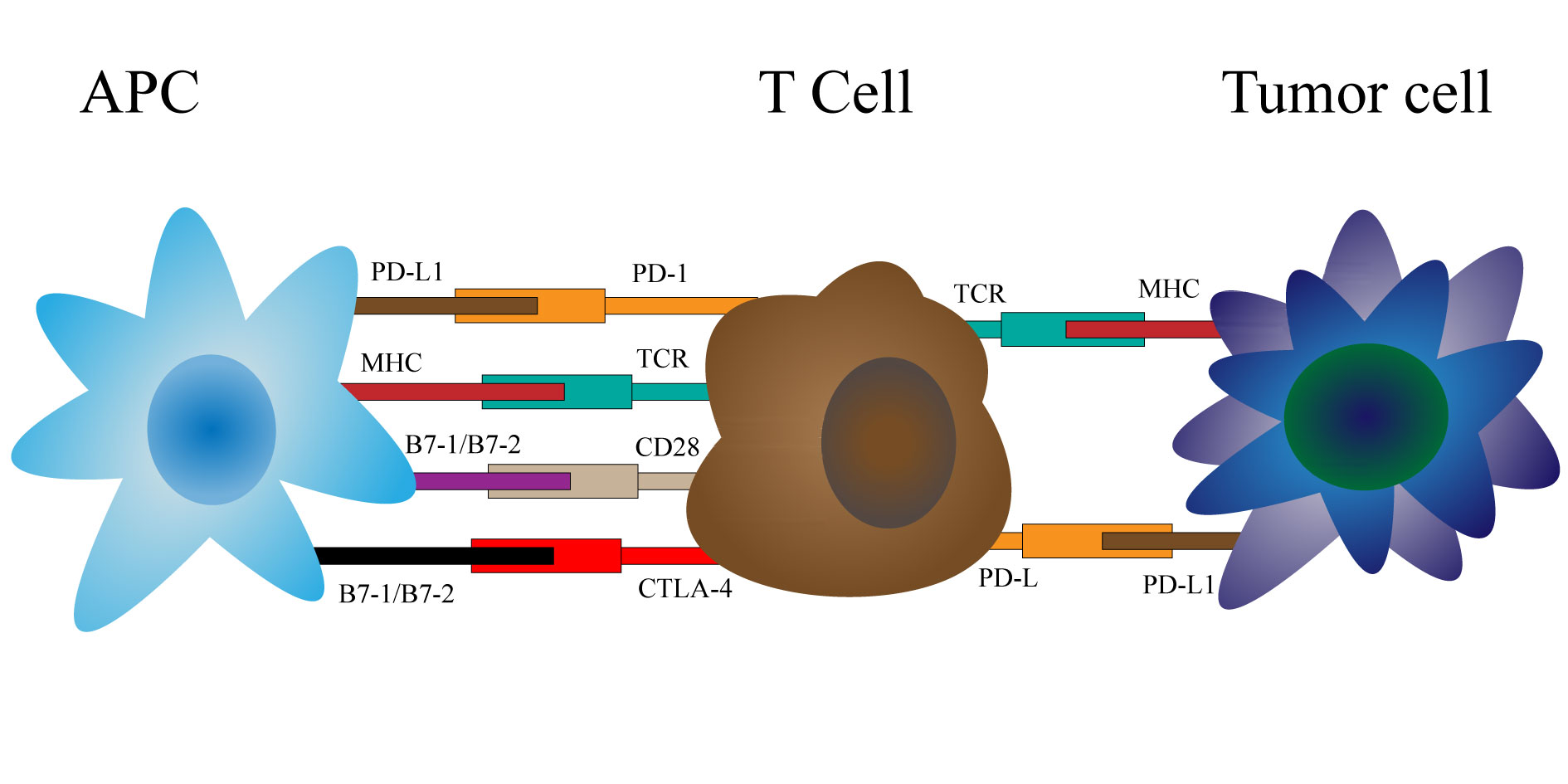
CTLA-4 expression in RCC
RCC is essentially a metabolic disease characterized by reprogramming of energy metabolism, and many of the genes mutated in RCC encode proteins that play a role in regulating cellular processes of oxygen and glucose consumption [38]. In particular, the metabolic flux is distributed through glycolysis [39-42], Mitochondrial bioenergetics and oxidative phosphorylation are impaired during lipid metabolism [40, 43, 44]. In addition, RCC is one of the most immunoinvasive tumors [45, 46]. Emerging evidence suggests that activation of specific metabolic pathways has a role in regulating angiogenic and inflammatory characteristics [47, 48]. VHL mutations in mRCC increase the transcriptional activity of its target genes (e.g VEGF, glucose transporter 1, and erythropoietin), independent of oxygen levels, promoting angiogenesis and immunosuppression [38]. The complexity of cell interactions and the depletion of available nutrients may create a nutrient-competitive environment for T cells and accumulate waste products that may damage T cells [49]. Rcc-bm exhibits metabolic changes resulting in altered pathways related to energy metabolism and oxidative stress, as well as accumulation of immunosuppressive metabolites such as tryptophan (TRP) [38, 49]. The enhanced activity of a series of interconnected oncogenic signaling networks centered on the PI3K-AKT pathway represents a generalizable feature in different BM histologies [49]. CTLA4 inhibits CD28 signaling and PI3K/Akt/mTORC1 signaling, resulting in decreased glycolysis and mitochondrial oxidative capacity [50]. Blocking the negative regulators of PD-1 and CTLA4, which impair CD28 signaling to inhibit T cell release, favors antitumor activity [38].
By survival analysis, it was found that high TII score had better prognosis than low TII score. GSEA analysis showed that the genes in high TII score group were rich in immunosuppressive pathways, such as ERBB signaling pathway, MAKP signaling pathway, mTOR signaling pathway and TGFβ signaling pathway [51]. A large number of clinical studies have confirmed that Anti-PD-1 and Anti-CTLA-4 antibodies can effectively inhibit the immune escape of cancer cells. Different from radiotherapy and chemotherapy drugs, the mechanism of immunotherapy is not to kill cancer cells directly, but to attack cancer cells indirectly by enhancing the specific anti-tumor cells of DC-CIK cells. Experimental study confirmed that the combination therapy can provide the synergistic anti-tumor effect of DC-CIK cells by suppressing proliferation, differentiation and early activation of RCC cells and regulating the immune stimulation and inhibiting the secretion of cytokines [52]. In RCC, about 1% of TIMCs express CTLA-4 [53] and the expression increased with the increase of tumor stage. In papillary RCC, up to 2.7% of TIMCs expressed CTLA-4 [54]. Polymorphisms in the CTLA-4 gene are associated with a higher risk of advanced ccRCC [55]. Allison and co-workers first demonstrated that administration of a CTLA-4 blocking antibody in mice prevented tumor establishment and induced rejection of established tumors [28].
Ipilimumab
Ipilimumab is a human IgG1 mAb that can inhibit the function of CTLA-4 and was first approved and recommended for the treatment of melanoma in 2011 [56]. It selectively blocks the interaction between the negative regulation of CTLA-4 on activated T cells and its ligands CD80/CD86 expressed on immune cells. In a phase 2 trial (NCT 00057889), the efficacy of ipilimumab monotherapy was evaluated in patients with metastatic RCC disease treated with ipilimumab high-dose (HD) (3 mg/kg every 3 weeks) versus low-dose (LD) (3 mg/kg once followed by 1 mg/kg every 3 weeks) [57]. Of the 40 patients treated with HD ipilimumab, 5 achieved PR (12.5%), including patients who had previously progressed on IL-2 therapy [57]. Of the 21 patients with LD, only 1 had PR.no CRs in either arm. Grade 3 or higher TRAEs (Treatment-related Adverse Events), particularly autoimmune-related enteritis and endocrine defects, were present in 33% of patients. The most common autoimmune events were enterocolitis (18%), hypophysitis (7%), and dermatitis (4%). No further studies have been conducted with ipilimumab monotherapy in RCC.
Tremelimumab
Tremelimumab is another anti-CTLA-4 monoclonal antibody. In phase 1 trial (NCT00372853) tremelimumab was administered intravenously to patients with mRCC at doses of 6, 10, or 15 mg/kg every 12 weeks in combination with sunitinib at 50 mg daily for 4 weeks, followed by 2 weeks or 37.5 mg daily [58]. Two of the five patients receiving tremelimumab 6 mg experienced unexpected rapid onset renal failure with sunitinib 50 mg, and one of the seven patients receiving tremelimumab 10 mg/kg plus sunitinib 37.5 mg died suddenly. The expansion cohort was treated with tremelimumab 10 mg/kg plus sunitinib 37.5 mg. However, dose-limiting toxicities were observed in three or seven patients. Of the nine patients evaluable, 43% achieved a partial response, but the regimen did not progress further due to toxicity.
Ipilimumab (anti-CTLA-4 antibody) and Nivolumab (anti-PD-1 antibody)
In a Phase 3 Checkmate 214 study in advanced RCC (NCT 02210117), 1096 patients were randomized to receive the combination of ipilimumab and nivolumab (n=550) and sunitinib (n=546). The co-primary endpoints of this study according to IMDC criteria were OS, PFS, and ORR (Objective Response Rate) in intermediate and low risk patients. ORR, PFS, and OS in the favorable risk group, and incidence of adverse events (AEs) in patients were secondary endpoints. In intermediate/low risk patients, combination therapy was associated with improved survival (HR: 0.63; 99.8% confidence interval: 0.44-0.89) and ORR (42 vs 27%), but no PFS reached the prespecified threshold (HR: 0.82; 99.1% confidence interval: 0.64-1.05). Of note, the combination of ipilimumab and nivolumab also improved the complete response rate (9 vs 1%). In patients at favorable risk, the combination did not result in a benefit in OS and ORR compared with sunitinib, however, complete response rates significantly favored the nivolumab and ipilimumab arms (11 vs 6%). There were 8 treatment-related deaths in the combination arm and 4 in the sunitinib arm. Increase in lipase, fatigue, diarrhea, rash, nausea, and decrease in appetite, and asthenia were the most common high-level AEs in the combination arm, while hypertension, palmar-plantar redness, fatigue, diarrhea, lipase increased, asthenia, vomiting, and anemia were the most common high-level AEs in the sunitinib arm. Despite this, the quality of life assessment showed that the combination arm was significantly better than the sunitinib arm [59, 60]. There was a statistically significant improvement in overall response rate (ORR) compared with sunitinib standard of care in first-line treatment of intermediate and low-risk patients [61]. However, nivolumab in combination with ipilimumab is approved as a representative first-line treatment for patients with mRCC at intermediate or low risk of IMDC [62]. Studies described in the above paragraph are summarized in Table 1.
Table 1. Immune checkpoint inhibitor-based adjuvant therapy trials in RCC. |
|||
Study |
N |
Description |
Primary Outcome to be Assessed |
NCT 00057889 |
61 |
Two sequential cohorts received either 3 mg/kg followed by 1 mg/kg or all doses at 3 mg/kg every 3 weeks |
A primary end point of response by Response Evaluation Criteria in Solid Tumors (RECIST) criteria |
NCT00372853 |
28 |
Tremelimumab (6 mg/kg, 10 mg/kg, or 15 mg/kg) intravenously once every 12 weeks and oral sunitinib (50 mg daily for 4 weeks then 2 weeks off or 37.5 mg daily as a continuous dose) |
The primary objective was to determine the maximum tolerated dose (MTD). Secondary objectives were to assess antitumor activity, safety, and pharmacokinetics |
NCT 02210117 |
1096 |
Nivolumab 3 mg/kg plus ipilimumab 1 mg/kg every 3 weeks for four doses followed by nivolumab 3 mg/kg every 2 weeks, or sunitinib 50 mg/day for 4 weeks of each 6-week cycle |
According to IMDC criteria, OS, PFS, and ORR in intermediate and low risk patients, ORR, PFS, and OS in the favorable risk group, and incidence of adverse events (AEs) in patients were secondary endpoints |
The first-line treatment modality for ccRCC has changed, particularly in intermediate/low risk patients, with the addition of nivolumab and ipilimumab in combination. However, more studies and better designed further studies are needed to explore the possibility and effectiveness of different drug combinations, while still focusing on their side effects, leading to better tumour outcomes. Future studies will likely identify biomarkers of subsequent immune response to better select candidates for neoadjuvant immunotherapy.
Acknowledgements
We thank Dr. Peng Zhang for his guidance and help. Thanks to our friends who helped us.
Ethical policy
All procedures performed in this study were in accordance with the ethical standards of the institutional and/or national research committee and with the 1964 Helsinki declaration and its later amendments or comparable ethical standards. Informed consent was obtained from all individual participants included in the study. Approval from institutional ethical committee was taken.
Availability of data and materials
All data generated or analysed during this study are included in this publication.
Author contributions
Juan Wu conceptualised, designed the study and was responsible for the writing of the original draft. Ya-fei Ren, Jun Xie and Dong-sheng Li reviewed and revised the manuscript. All authors approved the version of the manuscript to be submitted.
Competing interests
The authors declare no conflict of interest.
Funding
None.
- Siegel RL, Miller KD, Jemal A: Cancer statistics, 2018. CA Cancer J Clin 2018, 68(1): 7-30.
- Akhtar M, Al-Bozom IA, Al Hussain T: Papillary Renal Cell Carcinoma (PRCC): An Update. Adv Anat Pathol 2019, 26(2): 124-132.
- Siegel RL, Miller KD, Jemal A: Cancer Statistics, 2017. CA Cancer J Clin 2017, 67(1): 7-30.
- Choueiri TK, Motzer RJ: Systemic Therapy for Metastatic Renal-Cell Carcinoma. N Engl J Med 2017, 376(4): 354-366.
- Escudier B, Porta C, Schmidinger M, Algaba F, Patard JJ, Khoo V, Eisen T, Horwich A: Renal cell carcinoma: ESMO Clinical Practice Guidelines for diagnosis, treatment and follow-up. Ann Oncol 2014, 25 Suppl 3: iii49-56.
- Escudier B, Goupil MG, Massard C, Fizazi K: Sequential therapy in renal cell carcinoma. Cancer 2009, 115(10 Suppl): 2321-2326.
- Pal SK, Ghate SR, Li N, Swallow E, Peeples M, Zichlin ML, Perez JR, Agarwal N, Vogelzang NJ: Real-World Survival Outcomes and Prognostic Factors Among Patients Receiving First Targeted Therapy for Advanced Renal Cell Carcinoma: A SEER-Medicare Database Analysis. Clin Genitourin Cancer 2017, 15(4): e573-e582.
- Hanahan D, Weinberg RA: Hallmarks of cancer: the next generation. Cell 2011, 144(5): 646-674.
- Chang AJ, Zhao L, Zhu Z, Boulanger K, Xiao H, Wakefield MR, Bai Q, Fang Y: The Past, Present and Future of Immunotherapy for Metastatic Renal Cell Carcinoma. Anticancer Res 2019, 39(6): 2683-2687.
- Gleave ME, Elhilali M, Fradet Y, Davis I, Venner P, Saad F, Klotz LH, Moore MJ, Paton V, Bajamonde A: Interferon gamma-1b compared with placebo in metastatic renal-cell carcinoma. Canadian Urologic Oncology Group. N Engl J Med 1998, 338(18): 1265-1271.
- Hah YS, Koo KC: Immunology and Immunotherapeutic Approaches for Advanced Renal Cell Carcinoma: A Comprehensive Review. Int J Mol Sci 2021, 22(9).
- Robert C: A decade of immune-checkpoint inhibitors in cancer therapy. Nat Commun 2020, 11(1): 3801.
- Johnson DB, Nebhan CA, Moslehi JJ, Balko JM: Immune-checkpoint inhibitors: long-term implications of toxicity. Nat Rev Clin Oncol 2022, 19(4): 254-267.
- Tivol EA, Borriello F, Schweitzer AN, Lynch WP, Bluestone JA, Sharpe AH: Loss of CTLA-4 leads to massive lymphoproliferation and fatal multiorgan tissue destruction, revealing a critical negative regulatory role of CTLA-4. Immunity 1995, 3(5): 541-547.
- Freeman GJ, Long AJ, Iwai Y, Bourque K, Chernova T, Nishimura H, Fitz LJ, Malenkovich N, Okazaki T, Byrne MC et al: Engagement of the PD-1 immunoinhibitory receptor by a novel B7 family member leads to negative regulation of lymphocyte activation. J Exp Med 2000, 192(7): 1027-1034.
- Topalian SL, Hodi FS, Brahmer JR, Gettinger SN, Smith DC, McDermott DF, Powderly JD, Carvajal RD, Sosman JA, Atkins MB et al: Safety, activity, and immune correlates of anti-PD-1 antibody in cancer. N Engl J Med 2012, 366(26): 2443-2454.
- Schildberg FA, Klein SR, Freeman GJ, Sharpe AH: Coinhibitory Pathways in the B7-CD28 Ligand-Receptor Family. Immunity 2016, 44(5): 955-972.
- Ribas A, Wolchok JD: Cancer immunotherapy using checkpoint blockade. Science 2018, 359(6382): 1350-1355.
- Schwartz JC, Zhang X, Fedorov AA, Nathenson SG, Almo SC: Structural basis for co-stimulation by the human CTLA-4/B7-2 complex. Nature 2001, 410(6828): 604-608.
- Stamper CC, Zhang Y, Tobin JF, Erbe DV, Ikemizu S, Davis SJ, Stahl ML, Seehra J, Somers WS, Mosyak L: Crystal structure of the B7-1/CTLA-4 complex that inhibits human immune responses. Nature 2001, 410(6828): 608-611.
- Brunet JF, Denizot F, Luciani MF, Roux-Dosseto M, Suzan M, Mattei MG, Golstein P: A new member of the immunoglobulin superfamily--CTLA-4. Nature 1987, 328(6127): 267-270.
- Alegre ML, Noel PJ, Eisfelder BJ, Chuang E, Clark MR, Reiner SL, Thompson CB: Regulation of surface and intracellular expression of CTLA4 on mouse T cells. J Immunol 1996, 157(11): 4762-4770.
- Pardoll DM: The blockade of immune checkpoints in cancer immunotherapy. Nat Rev Cancer 2012, 12(4): 252-264.
- Rotte A: Combination of CTLA-4 and PD-1 blockers for treatment of cancer. J Exp Clin Cancer Res 2019, 38(1): 255.
- Fife BT, Bluestone JA: Control of peripheral T-cell tolerance and autoimmunity via the CTLA-4 and PD-1 pathways. Immunol Rev 2008, 224: 166-182.
- Wing K, Onishi Y, Prieto-Martin P, Yamaguchi T, Miyara M, Fehervari Z, Nomura T, Sakaguchi S: CTLA-4 control over Foxp3+ regulatory T cell function. Science 2008, 322(5899): 271-275.
- Krummel MF, Allison JP: CD28 and CTLA-4 have opposing effects on the response of T cells to stimulation. J Exp Med 1995, 182(2): 459-465.
- Leach DR, Krummel MF, Allison JP: Enhancement of antitumor immunity by CTLA-4 blockade. Science 1996, 271(5256): 1734-1736.
- Chambers CA, Sullivan TJ, Allison JP: Lymphoproliferation in CTLA-4-deficient mice is mediated by costimulation-dependent activation of CD4+ T cells. Immunity 1997, 7(6): 885-895.
- Intlekofer AM, Thompson CB: At the bench: preclinical rationale for CTLA-4 and PD-1 blockade as cancer immunotherapy. J Leukoc Biol 2013, 94(1): 25-39.
- Chikuma S, Abbas AK, Bluestone JA: B7-independent inhibition of T cells by CTLA-4. J Immunol 2005, 175(1): 177-181.
- Schneider H, Valk E, Leung R, Rudd CE: CTLA-4 activation of phosphatidylinositol 3-kinase (PI 3-K) and protein kinase B (PKB/AKT) sustains T-cell anergy without cell death. PLoS One 2008, 3(12): e3842.
- Fraser JH, Rincón M, McCoy KD, Le Gros G: CTLA4 ligation attenuates AP-1, NFAT and NF-kappaB activity in activated T cells. Eur J Immunol 1999, 29(3): 838-844.
- Bhandaru M, Rotte A: Monoclonal Antibodies for the Treatment of Melanoma: Present and Future Strategies. Methods Mol Biol 2019, 1904: 83-108.
- Castillero F, Castillo-Fernández O, Jiménez-Jiménez G, Fallas-Ramírez J, Peralta-?lvarez MP, Arrieta O: Cancer immunotherapy-associated hypophysitis. Future Oncol 2019, 15(27): 3159-3169.
- Faje A: Immunotherapy and hypophysitis: clinical presentation, treatment, and biologic insights. Pituitary 2016, 19(1): 82-92.
- Arce Vargas F, Furness AJS, Litchfield K, Joshi K, Rosenthal R, Ghorani E, Solomon I, Lesko MH, Ruef N, Roddie C et al: Fc Effector Function Contributes to the Activity of Human Anti-CTLA-4 Antibodies. Cancer Cell 2018, 33(4): 649-663.e644.
- Beckermann KE, Hongo R, Ye X, Young K, Carbonell K, Healey DCC, Siska PJ, Barone S, Roe CE, Smith CC et al: CD28 costimulation drives tumor-infiltrating T cell glycolysis to promote inflammation. JCI Insight 2020, 5(16).
- Lucarelli G, Loizzo D, Franzin R, Battaglia S, Ferro M, Cantiello F, Castellano G, Bettocchi C, Ditonno P, Battaglia M: Metabolomic insights into pathophysiological mechanisms and biomarker discovery in clear cell renal cell carcinoma. Expert Rev Mol Diagn 2019, 19(5): 397-407.
- Bianchi C, Meregalli C, Bombelli S, Di Stefano V, Salerno F, Torsello B, De Marco S, Bovo G, Cifola I, Mangano E et al: The glucose and lipid metabolism reprogramming is grade-dependent in clear cell renal cell carcinoma primary cultures and is targetable to modulate cell viability and proliferation. Oncotarget 2017, 8(69): 113502-113515.
- Ragone R, Sallustio F, Piccinonna S, Rutigliano M, Vanessa G, Palazzo S, Lucarelli G, Ditonno P, Battaglia M, Fanizzi FP et al: Renal Cell Carcinoma: A Study through NMR-Based Metabolomics Combined with Transcriptomics. Diseases 2016, 4(1).
- Lucarelli G, Galleggiante V, Rutigliano M, Sanguedolce F, Cagiano S, Bufo P, Lastilla G, Maiorano E, Ribatti D, Giglio A et al: Metabolomic profile of glycolysis and the pentose phosphate pathway identifies the central role of glucose-6-phosphate dehydrogenase in clear cell-renal cell carcinoma. Oncotarget 2015, 6(15): 13371-13386.
- Lucarelli G, Rutigliano M, Sallustio F, Ribatti D, Giglio A, Lepore Signorile M, Grossi V, Sanese P, Napoli A, Maiorano E et al: Integrated multi-omics characterization reveals a distinctive metabolic signature and the role of NDUFA4L2 in promoting angiogenesis, chemoresistance, and mitochondrial dysfunction in clear cell renal cell carcinoma. Aging (Albany NY) 2018, 10(12): 3957-3985.
- Bombelli S, Torsello B, De Marco S, Lucarelli G, Cifola I, Grasselli C, Strada G, Bovo G, Perego RA, Bianchi C: 36-kDa Annexin A3 Isoform Negatively Modulates Lipid Storage in Clear Cell Renal Cell Carcinoma Cells. Am J Pathol 2020, 190(11): 2317-2326.
- Vuong L, Kotecha RR, Voss MH, Hakimi AA: Tumor Microenvironment Dynamics in Clear-Cell Renal Cell Carcinoma. Cancer Discov 2019, 9(10): 1349-1357.
- Tamma R, Rutigliano M, Lucarelli G, Annese T, Ruggieri S, Cascardi E, Napoli A, Battaglia M, Ribatti D: Microvascular density, macrophages, and mast cells in human clear cell renal carcinoma with and without bevacizumab treatment. Urol Oncol 2019, 37(6): 355.e311-355.e319.
- Netti GS, Lucarelli G, Spadaccino F, Castellano G, Gigante M, Divella C, Rocchetti MT, Rascio F, Mancini V, Stallone G et al: PTX3 modulates the immunoflogosis in tumor microenvironment and is a prognostic factor for patients with clear cell renal cell carcinoma. Aging (Albany NY) 2020, 12(8): 7585-7602.
- Lucarelli G, Rutigliano M, Ferro M, Giglio A, Intini A, Triggiano F, Palazzo S, Gigante M, Castellano G, Ranieri E et al: Activation of the kynurenine pathway predicts poor outcome in patients with clear cell renal cell carcinoma. Urol Oncol 2017, 35(7): 461.e415-461.e427.
- Fukumura K, Malgulwar PB, Fischer GM, Hu X, Mao X, Song X, Hernandez SD, Zhang XH, Zhang J, Parra ER et al: Multi-omic molecular profiling reveals potentially targetable abnormalities shared across multiple histologies of brain metastasis. Acta Neuropathol 2021, 141(2): 303-321.
- Patsoukis N, Bardhan K, Chatterjee P, Sari D, Liu B, Bell LN, Karoly ED, Freeman GJ, Petkova V, Seth P et al: PD-1 alters T-cell metabolic reprogramming by inhibiting glycolysis and promoting lipolysis and fatty acid oxidation. Nat Commun 2015, 6: 6692.
- Bai D, Feng H, Yang J, Yin A, Qian A, Sugiyama H: Landscape of immune cell infiltration in clear cell renal cell carcinoma to aid immunotherapy. Cancer Sci 2021, 112(6): 2126-2139.
- Yuan Z, Yang H, Wei Y: Combined induction with anti-PD-1 and anti-CTLA-4 antibodies provides synergistic antitumor effects in DC-CIK cells in renal carcinoma cell lines. Int J Clin Exp Pathol 2019, 12(1): 123-132.
- Kahlmeyer A, St?hr CG, Hartmann A, Goebell PJ, Wullich B, Wach S, Taubert H, Erlmeier F: Expression of PD-1 and CTLA-4 Are Negative Prognostic Markers in Renal Cell Carcinoma. J Clin Med 2019, 8(5): 743.
- Geissler K, Fornara P, Lautenschl?ger C, Holzhausen HJ, Seliger B, Riemann D: Immune signature of tumor infiltrating immune cells in renal cancer. Oncoimmunology 2015, 4(1): e985082.
- Tupikowski K, Partyka A, Kolodziej A, Dembowski J, Debinski P, Halon A, Zdrojowy R, Frydecka I, Karabon L: CTLA-4 and CD28 genes' polymorphisms and renal cell carcinoma susceptibility in the Polish population--a prospective study. Tissue Antigens 2015, 86(5): 353-361.
- Callahan MK, Wolchok JD: At the bedside: CTLA-4- and PD-1-blocking antibodies in cancer immunotherapy. J Leukoc Biol 2013, 94(1): 41-53.
- Yang JC, Hughes M, Kammula U, Royal R, Sherry RM, Topalian SL, Suri KB, Levy C, Allen T, Mavroukakis S et al: Ipilimumab (anti-CTLA4 antibody) causes regression of metastatic renal cell cancer associated with enteritis and hypophysitis. J Immunother 2007, 30(8): 825-830.
- Rini BI, Stein M, Shannon P, Eddy S, Tyler A, Stephenson JJ, Jr., Catlett L, Huang B, Healey D, Gordon M: Phase 1 dose-escalation trial of tremelimumab plus sunitinib in patients with metastatic renal cell carcinoma. Cancer 2011, 117(4): 758-767.
- Cella D, Grünwald V, Escudier B, Hammers HJ, George S, Nathan P, Grimm MO, Rini BI, Doan J, Ivanescu C et al: Patient-reported outcomes of patients with advanced renal cell carcinoma treated with nivolumab plus ipilimumab versus sunitinib (CheckMate 214): a randomised, phase 3 trial. Lancet Oncol 2019, 20(2): 297-310.
- Massari F, Di Nunno V: CheckMate 214 patient-reported outcomes: listening to our patients. Lancet Oncol 2019, 20(2): 179-180.
- Motzer RJ, Tannir NM, McDermott DF, Arén Frontera O, Melichar B, Choueiri TK, Plimack ER, Barthélémy P, Porta C, George S et al: Nivolumab plus Ipilimumab versus Sunitinib in Advanced Renal-Cell Carcinoma. N Engl J Med 2018, 378(14): 1277-1290.
- Gao X, McDermott DF: Ipilimumab in combination with nivolumab for the treatment of renal cell carcinoma. Expert Opin Biol Ther 2018, 18(9): 947-957.
- Massari F, Nunno VD, Mollica V, Montironi R, Cheng L, Cimadamore A, Blanca A, Lopez-Beltran A: Immunotherapy in renal cell carcinoma from poverty to the spoiled of choice. Immunotherapy 2019, 11(17): 1507-1521.
- Peinemann F, Unverzagt S, Hadjinicolaou AV, Moldenhauer I: Immunotherapy for metastatic renal cell carcinoma: A systematic review. J Evid Based Med 2019, 12(4): 253-262.
Annals of urologic oncology
p-ISSN: 2617-7765, e-ISSN: 2617-7773
Copyright © Ann Urol Oncol. This work is licensed under a Creative Commons Attribution-NonCommercial-No Derivatives 4.0 International (CC BY-NC-ND 4.0) License.