Review Article | Open Access
Research Progression in the Mechanism of Bone Metastasis and Bone-Targeted Drugs in Prostate Cancer
Sajjad Ahmad11Department of Health and Biological Sciences, Abasyn University, Peshawar, Pakistan.
Correspondence: Sajjad Ahmad (Department of Health and Biological Sciences, Abasyn University, Ring Road Kabothar Chock, Peshawar, Pakistan; sajjad.ahmad@abasyn.edu.pk).
Annals of Urologic Oncology 2024, 7(1): 18-25. https://doi.org/10.32948/auo.2024.02.20
Received: 28 Jan 2024 | Accepted: 20 Feb 2024 | Published online: 24 Feb 2024
Key words prostate cancer, bone metastasis mechanism, bone-targeted drug
Fixation refers to the process by which cancer cells enter the bone marrow cavity through the circulatory system. The cytokines synthesized during bone matrix release and bone turnover promote the colonization of prostate cancer cells in the bone [16-18]. Studies have demonstrated that chemokine and receptor interactions play an important role in bone metastasis of prostate cancer. The increase of chemokine ligand 12 (C-X-C motif chemokine ligand 12, CXCL12) in bone tissue is associated with tumor metastasis. CXCL12 binds to C-X-Cmotif chemokine receptor 4 (CXCR4) to promote the adhesion, invasion, and migration of prostate cancer cells [19]. CXCR4 is expressed by prostate cancer cells and binding to CXCL12 promotes the colonization and deposition of prostate cancer cells in bone tissue [20]. Studies have shown that knockdown of androgen receptor (AR) signals in tumor-associated fibroblasts (CAFs), significantly increases the expression of Chemokine ligand 2 (CCL2), which can enhance the migration ability of prostate cancer cells [21-23]. Growth differentiation factor 15 activates osteoclast generation through CCL2 and a Receptor activator of nuclear factor-κB ligand (RANKL) produced by osteoblasts increases osteoblast function and promotes bone metastasis and colonization [24-26]. The chemokine receptor CXCR2 stimulates the secretion of vascular endothelial growth factor (VEGF), promotes the formation of pre-metastatic niche in bone, and enhances the homing effect of prostate cancer cells to bone [27, 28]. Integrin is regulated by a variety of cytokines and plays a role in modifying the cytoskeleton and increasing the metastatic ability of prostate cancer. After CXCL16 binds to its specific receptor CXCR6, integrin αvβ3 accumulates in the front of the cell membrane, driving the change of tumor cytoskeleton dynamics and increasing the migration, invasion, and adhesion ability of endothelial cells [29]. Integrin αv is enriched in bone metastases of prostate cancer, integrin α5 is specifically expressed in bone metastases tumor stroma and endothelial cells, but not in primary tumors, and the abnormal expression of both in primary and bone metastases is consistent with bone colonization [30]. Figure 1 shows that increase of CXCL12 in bone tissue is associated with tumor metastasis.
The dormancy of tumor cells
Dormant diffuse tumor cells can often develop into secondary tumors of bone, and dormant cells are often resistant to conventional chemotherapeutic drugs, preventing drug clearance from tumor cells [31, 32]. After bone metastasis of prostate cancer, dormant cancer cells congregate around osteoblasts and expresses high levels of tyrosine kinase receptors, which were involved in regulating the expression of transforming growth factor β (TGF-β) and its receptors [33]. TGF-β2 is secreted by bone marrow stromal cells, which can up-regulate the expression level of growth arrest-specific protein (GAS protein) 6. One of the steps of prostate cancer cell dormancy regulation is the specific binding of GAS protein to AXL. Therefore, it is theorized that specifically blocking the TGF-β signaling pathway can limit osteoblast-induced dormancy of prostate cancer cells [33]. Bone morphogenetic protein 7 (BMP7) can activate p38 mitogen-activated protein kinase, increase the expression of cell cycle inhibitor p21 and metastasis suppressor gene NDRG1, and induce prostate cancer stem-like cell senescence. It plays an important role in the dormancy and recurrence of prostate cancer [34]. Further studies showed that RANKL secreted by bone cells could be correlated with the Receptor activator of nuclear factor-κB, which is highly expressed in prostate cancer. RANK specifically binds to promote EMT of prostate cancer cells and up-regulates the expression of the Wnt signaling pathway in malignant cells [35, 36]. The Wnt /β catenin signaling pathway is related to the dormancy of prostate cancer. Wnt5α, as an important member of this pathway, induces and maintains the dormancy of prostate cancer cells in bone through the Wnt5α /ROR2 /SIAH2 signaling axis [37-41].
Reactivation of dormant cells
Dormant prostate cancer cells can be activated to induce proliferative state by specific factors, such as cell adhesion molecules, hypoxia in the bone microenvironment, and angiogenesis [17]. Once colonized dormant tumor cells are reactivated, they will continue to proliferate and infiltrate. It has been found that the extracellular matrix in the tumor microenvironment promotes the formation of blood vessels, and bone resorption caused by osteoclasts, and changes in the immune escape state of tumor cells can cause reactivation of prostate cancer cells [42]. Lawson et al. [43] found that osteoblasts and osteoclasts in the bone microenvironment can have the ability to promote and relieve tumor cell dormancy. It was found that sustained inflammation induced neutrophils to form an extracellular snare NET (NET), and two NET-related proteases (neutrophil elastase and matrix metalloproteinase-9) lysed laminin. Laminin after proteolytic remodeling induces the proliferation of dormant prostate cancer cells by activating integrin α3β1 signaling [44].In addition, down-regulation of BMP-7 expression may result in inhibition of PC-3 cell dormancy. Therefore, osteoclast activation, Gas6, and BMP-7 are key players in regulating the dormancy reactivation of tumor cells [33]. CXCL8 is rapidly induced by tumor necrosis factor α(TNF-α) and IL-1β, and promotes tumor cell proliferation and angiogenesis through the CXCL8 /CXCR1 and CXCL8 /CXCR2 axes [45]. VEGF binding to the tyrosine kinase receptor VEGF2 expressed by endothelial cells can stimulate angiogenesis, and integrin αvβ3 can significantly enhance the expression of this signaling pathway, further stimulate downstream FAK/SrC /Paxillin signal activation, and promote the participation of ERK1/2 and PI3K pathways in angiogenesis [46]. In addition, it was found that norepinephrine (NE)) plays an important role in the reactivation of dormant prostate cancer cells. Through β2 adrenergic receptors, NE can directly stimulate the proliferation of prostate cancer cells and indirectly down-regulate the secretion of GAS6 to inhibit cell dormancy [47].
Reconstruction of metastatic bone in prostate cancer
The activated tumor cells can enter the proliferative stage under the stimulation of various factors in the bone microenvironment. After metastasis to bone, the homeostasis between osteoblasts and osteoclasts is destroyed. In particular, the destruction of osteoblasts can form a "vicious cycle" to promote tumor cell growth, continuous osteoclast generation, and bone absorption. Bone metastasis in prostate cancer changes the balance between osteoclast absorption and osteoblast formation, and reconstructs the original bone structure and function, which is mainly manifested as osteoblastosis, accelerates the original bone injury at the end of metastasis, and forms the woven bone with random arrangement of collagen fibers. Related cytokines secreted by woven bone stromal cells and bone cells can further promote the growth of malignant cells [48, 49]. Prostate cancer cells secrete bone morphogenetic protein-4 to promote the transformation of bone marrow endothelial cells into osteoblasts, and prostate-specific antigen (PSA) can also participate in the formation of osteoblasts through prostate-specific antigen (PSA) cleavage of parathyroid hormone-related peptides [50]. In osteoblasts, RANKL can promote osteoclast generation after binding with RANK receptor on osteoclast precursor cells, while mature osteoblasts will produce osteoprotegerin (OPG), which competes with RANK receptor of osteoclasts to bind RANKL and inhibit osteoclast maturation [51]. Therefore, the formation of osteoclasts is co-regulated by the expression levels of RANKL and OPG. After osteoclasts degrade bone matrix, TGF-β is released, and TGF-β is acetylated by inducing transcription factor KLF5 (Kruppel-like factor). Activation of CXCR4 stimulates osteoclast formation and related bone metastases [52]. Integrin αvβ3 is expressed on prostate cancer cells and osteoclasts, and RANKL expression is involved in bone metastasis and bone resorption of prostate cancer. Studies have shown that periosteum protein is highly expressed in both osteoblasts and prostate cancer cells, that osteoblast-derived periosteum protein promotes proliferation of prostate cancer cells, and that the periosteum protein from prostate cancer cells also promotes the proliferation of osteoblasts, and that periosteum protein regulates the function of prostate cancer and osteoblasts through integrin receptors [53].
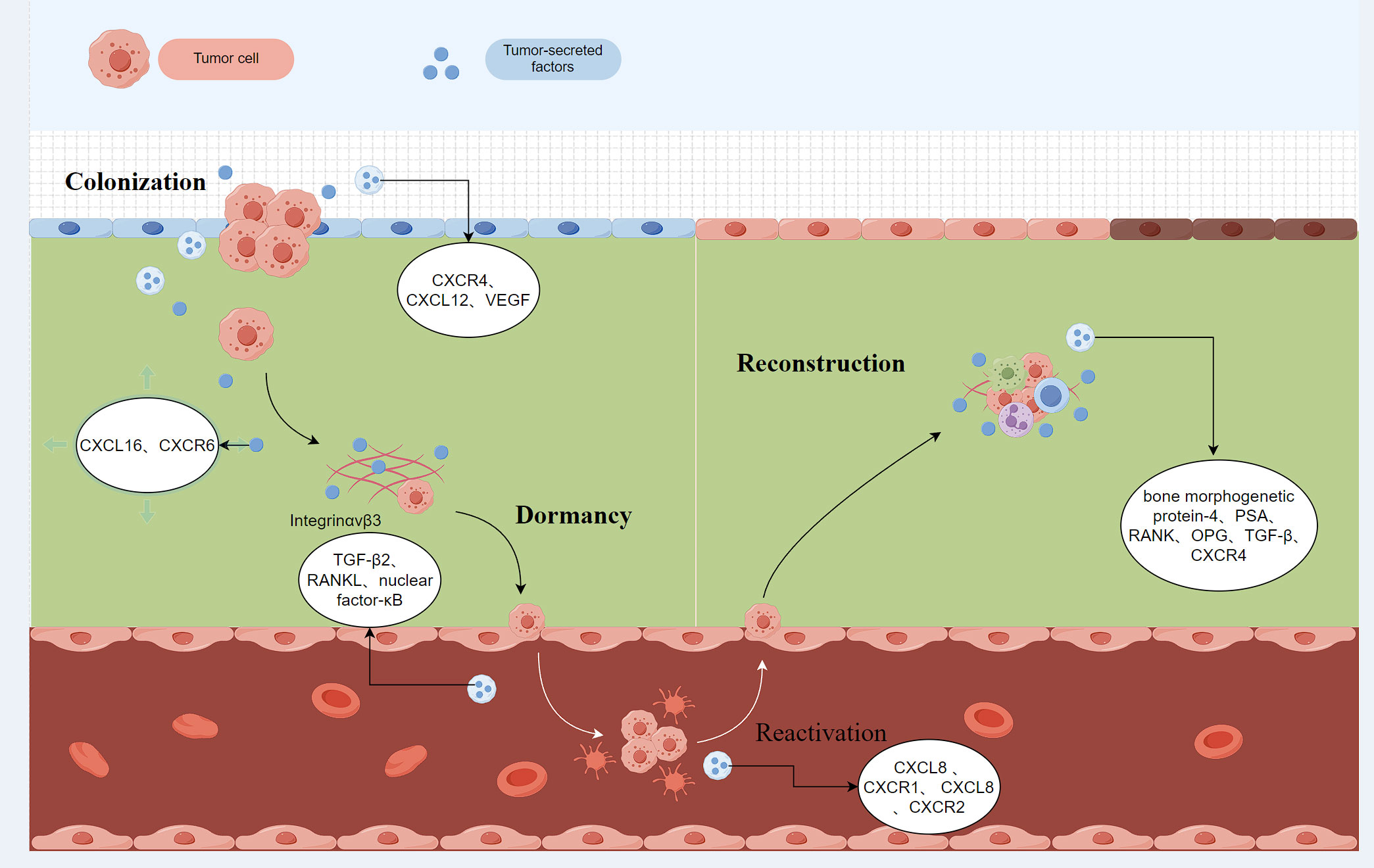
Due to the high affinity between bisphosphonates and bone tissue, zoledronic acid (ZA) was first approved by the US Food and Drug Administration for castration-resistant prostate cancer (CRPC )in 2022. Skeletal related events (SREs), which is currently the most effective nitrogenous bisphosphateis currently in use in the clinic [54-58]. ZA can not only reduce the number of osteoclasts but also inhibit the fusion of more mature osteoclast precursor cells on the bone surface while having little effect on osteoblasts. In vivo and in vitro models, ZA exerts antitumor activity by influencing apoptosis, and inhibiting tumor cell growth, adhesion, invasion, and angiogenesis [55]. Landgraf et al. [59, 60] found that after humanizing mouse bone to simulate the human bone microenvironment, prostate cancer cells were injected into the left ventricle of mice to simulate the bone metastasis process. At the 13th to 14th week, ZA showed an obvious inhibitory effect on osteoclasts, reducing the initial tumor load to 0. 78 and 0. 87 showing a significant therapeutic effect.
RANKL inhibitors
There are many types of fully human monoclonal antibodies, one of which, denosumab, was approved in Europe and the United States in 2011 for bone protection in patients at high risk of fracture due to bone metastases of solid tumors such as prostate cancer [61-66]. As a RANKL inhibitor, denosumab can bind to RANKL in the extracellular space in a unique way, achieving the purpose of inhibiting the binding of RANKL-Rank, blocking the activation of osteoclasts and reducing osteoclast-mediated bone resorption [67]. A meta-analysis comparing the efficacy of denosumab and ZA in the treatment of bone metastases in prostate cancer showed that the effect of denosumab on pain relief in patients with bone metastases was stronger than that in ZA group, and the rate of kidney injury was lower than that in ZA group. Unfortunately, denosumab had no significant effect on the overall survival of patients [68]. In a randomized, double-blind trial, 950 and 951 patients with CRPC bone metastases were treated with denosumab (120 mg /4 weeks, subcutaneous injection) and ZA (4 mg /4 weeks, intravenous injection), respectively. The median time for first SREs in patients treated with denosumab was 3. At 6 months, however, the rate of hypocalcemia in the denosumab group (121 cases, 13%) was higher than that in the ZA group (55 cases, 6%), and medical workers could reasonably use calcium in treatment to prevent the occurrence of related complications [56].
Radium-223,223Ra
223Ra is a calcium analogue that is absorbed into bone by osteoblasts through the same pathway as calcium, and decays to produce alpha particles after binding with bone, causing cytotoxic double-strand breaks (DSBs) in neighboring tumor cells and inducing apoptosis [69-73]. In the phase III clinical trial ALSYMPCA [74], patients treated with 223Ra had an average of 3.6 months better overall survival (OS) than those treated with placebo, and patients treated with 223Ra had a significant reduction in SREs and demonstrated a better quality of life. 223Ra is approved by the FDA for the treatment of mCRPC patients with bone-related symptoms or localized bone metastases and is the only drug found to improve metastatic castration-resistant prostate cancer. The European MedicineAgency (EMA) has also approved 223Ra for the treatment of patients with bone metastases who have had two mCRPC bone treatments or are unable to receive other treatment options [75].
Strontium-89, 89Sr
89Sr and 223Ra belong to the same calcium analogs and have the same principle of killing cancer cells. Compared with 223Ra, the β particles produced by the decay of 89Sr after absorption into bone have lower energy and wider radiation range than α particles, which may make the destruction effect of 89Sr on metastatic bone tumors lower than that of 223Ra. It's also more likely to cause damage to normal bone tissue [75-78]. The combination of 89Sr and ZA has been widely used for bone metastasis of lung cancer. The study found that the combination of the two drugs can improve the one-year survival rate of patients and delay the occurrence of SREs [79]. In recent years, it has been found that the combination of 89Sr and ZA can play a palliative effect on the pain caused by bone metastasis of prostate cancer [80, 81]. However, none of the conclusions can prove that 89Sr alone can benefit OS and SREs in patients with advanced bone metastasis. There are still few studies on 89Sr in CRPC bone metastasis, and further exploration is needed [82-84].
Samarium-153, 153 Sm
None.
Ethical policy
All procedures performed in this study were in accordance with the ethical standards of the institutional and/or national research committee and with the 1964 Helsinki declaration and its later amendments or comparable ethical standards. Informed consent was obtained from all individual participants included in the study. Approval from institutional ethical committee was taken.
Availability of data and materials
All data generated or analysed during this study are included in this publication.
Author contributions
SA: conception, design of study, literature search and review, manuscript writting, approval for the final version of the manuscript.
Competing interests
The authors have no competing interest.
Funding
None.
- Sung H, Ferlay J, Siegel RL: Global Cancer Statistics 2020: GLOBOCAN Estimates of Incidence and Mortality Worldwide for 36 Cancers in 185 Countries. CA Cancer J Clin 2021, 71(3): 209-249.
- Erratum: Global cancer statistics 2018: GLOBOCAN estimates of incidence and mortality worldwide for 36 cancers in 185 countries. CA Cancer J Clin 2020, 70(4): 313.
- Nuhn P, De Bono JS, Fizazi K, Freedland SJ, Grilli M, Kantoff PW, Sonpavde G, Sternberg CN, Yegnasubramanian S, Antonarakis ES: Update on Systemic Prostate Cancer Therapies: Management of Metastatic Castration-resistant Prostate Cancer in the Era of Precision Oncology. Eur Urol 2019, 75(1): 88-99.
- Teo MY, Rathkopf DE, Kantoff P: Treatment of Advanced Prostate Cancer. Annu Rev Med 2019, 70: 479-499.
- Eigl BJ, Gleave ME, Chi KN: The future of systemic therapies for localised prostate cancer. Clin Oncol (R Coll Radiol) 2013, 25(8): 506-513.
- Kang J, La Manna F, Bonollo F, Sampson N, Alberts IL, Mingels C, Afshar-Oromieh A, Thalmann GN, Karkampouna S: Tumor microenvironment mechanisms and bone metastatic disease progression of prostate cancer. Cancer Lett 2022, 530: 156-169.
- Li XF, Selli C: Macrophages promote anti-androgen resistance in prostate cancer bone disease. J Exp Med 2023, 220(4): e20221007.
- Hiraga T: Bone metastasis: Interaction between cancer cells and bone microenvironment. J Oral Biosci 2019, 61(2): 95-98.
- Singh DK, Patel VG, Oh WK: Prostate Cancer Dormancy and Reactivation in Bone Marrow. J Clin Med 2021, 10(12): 2648.
- Jung Y, Cackowski FC, Yumoto K, Decker AM, Wang Y, Hotchkin M, Lee E, Buttitta L, Taichman RS: Abscisic acid regulates dormancy of prostate cancer disseminated tumor cells in the bone marrow. Neoplasia 2021, 23(1): 102-111.
- Gartrell BA, Coleman R, Efstathiou E, Fizazi K, Logothetis CJ, Smith MR, Sonpavde G, Sartor O, Saad F: Metastatic Prostate Cancer and the Bone: Significance and Therapeutic Options. Eur Urol 2015, 68(5): 850-858.
- Poeppel TD, Handkiewicz-Junak D, Andreeff M, Becherer A, Bockisch A, Fricke E, Geworski L, Heinzel A, Krause BJ, Krause T et al: EANM guideline for radionuclide therapy with radium-223 of metastatic castration-resistant prostate cancer. Eur J Nucl Med Mol Imaging 2018, 45(5): 824-845.
- Fragkoulis C, Gkialas I, Papadopoulos G, Ntoumas K: Current therapeutic options targeting bone metastasis in metastatic castration resistant prostate cancer. J BUON 2016, 21(4): 787-791.
- von Moos R, Sternberg C, Body JJ, Bokemeyer C: Reducing the burden of bone metastases: current concepts and treatment options. Support Care Cancer 2013, 21(6): 1773-1783.
- Drake CG, Sharma P, Gerritsen W: Metastatic castration-resistant prostate cancer: new therapies, novel combination strategies and implications for immunotherapy. Oncogene 2014, 33(43): 5053-5064.
- Muralidhar A, Potluri HK, Jaiswal T, McNeel DG: Targeted Radiation and Immune Therapies-Advances and Opportunities for the Treatment of Prostate Cancer. Pharmaceutics 2023, 15(1): 252.
- Zhang X: Interactions between cancer cells and bone microenvironment promote bone metastasis in prostate cancer. Cancer Commun (Lond) 2019, 39(1): 76.
- Deng X, He G, Liu J, Luo F, Peng X, Tang S, Gao Z, Lin Q, Keller JM, Yang T et al: Recent advances in bone-targeted therapies of metastatic prostate cancer. Cancer Treat Rev 2014, 40(6): 730-738.
- Baci D, Bruno A, Cascini C, Gallazzi M, Mortara L, Sessa F, Pelosi G, Albini A, Noonan DM: Acetyl-L-Carnitine downregulates invasion (CXCR4/CXCL12, MMP-9) and angiogenesis (VEGF, CXCL8) pathways in prostate cancer cells: rationale for prevention and interception strategies. J Exp Clin Cancer Res 2019, 38(1): 464.
- Midavaine É, Côté J, Sarret P: The multifaceted roles of the chemokines CCL2 and CXCL12 in osteophilic metastatic cancers. Cancer Metastasis Rev 2021, 40(2): 427-445.
- Cioni B, Nevedomskaya E, Melis MHM, van Burgsteden J, Stelloo S, Hodel E, Spinozzi D, de Jong J, van der Poel H, de Boer JP et al: Loss of androgen receptor signaling in prostate cancer-associated fibroblasts (CAFs) promotes CCL2- and CXCL8-mediated cancer cell migration. Mol Oncol 2018, 12(8): 1308-1323.
- Sahai E, Astsaturov I: A framework for advancing our understanding of cancer-associated fibroblasts. Nat Rev Cancer 2020, 20(3): 174-186.
- Chen Y, McAndrews KM: Clinical and therapeutic relevance of cancer-associated fibroblasts. Nat Rev Clin Oncol 2021, 18(12): 792-804.
- Siddiqui JA, Seshacharyulu P: GDF15 promotes prostate cancer bone metastasis and colonization through osteoblastic CCL2 and RANKL activation. Bone Res 2022, 10(1): 6.
- Wang W, Yang X, Dai J, Lu Y, Zhang J, Keller ET: Prostate cancer promotes a vicious cycle of bone metastasis progression through inducing osteocytes to secrete GDF15 that stimulates prostate cancer growth and invasion. Oncogene 2019, 38(23): 4540-4559.
- Mimeault M, Johansson SL, Batra SK: Pathobiological implications of the expression of EGFR, pAkt, NF-κB and MIC-1 in prostate cancer stem cells and their progenies. PLoS One 2012, 7(2): e31919.
- Li Y, He Y: Targeting cellular heterogeneity with CXCR2 blockade for the treatment of therapy-resistant prostate cancer. Sci Transl Med 2019, 11(521): eaax0428.
- Ruiz de Porras V, Wang XC, Palomero L, Marin-Aguilera M, Solé-Blanch C, Indacochea A, Jimenez N, Bystrup S, Bakht M, Conteduca V et al: Taxane-induced Attenuation of the CXCR2/BCL-2 Axis Sensitizes Prostate Cancer to Platinum-based Treatment. Eur Urol 2021, 79(6): 722-733.
- Singh R, Kapur N, Mir H, Singh N, Lillard JW Jr, Singh S: CXCR6-CXCL16 axis promotes prostate cancer by mediating cytoskeleton rearrangement via Ezrin activation and αvβ3 integrin clustering. Oncotarget 2016, 7(6): 7343-7353.
- Connell B, Kopach P, Ren W, Joshi R, Naber S, Zhou M, Mathew P: Aberrant integrin αv and α5 expression in prostate adenocarcinomas and bone-metastases is consistent with a bone-colonizing phenotype. Transl Androl Urol 2020, 9(4): 1630-1638.
- Quayle L, Ottewell PD, Holen I: Bone Metastasis: Molecular Mechanisms Implicated in Tumour Cell Dormancy in Breast and Prostate Cancer. Curr Cancer Drug Targets 2015, 15(6): 469-480.
- Ban J, Fock V, Aryee DNT, Kovar H: Mechanisms, Diagnosis and Treatment of Bone Metastases. Cell 2021, 10(11): 2944.
- Yumoto K, Eber MR, Wang J, Cackowski FC, Decker AM, Lee E, Nobre AR, Aguirre-Ghiso JA, Jung Y, Taichman RS: Axl is required for TGF-β2-induced dormancy of prostate cancer cells in the bone marrow. Sci Rep 2016, 6: 36520.
- Kobayashi A, Okuda H, Xing F, Pandey PR, Watabe M, Hirota S, Pai SK, Liu W, Fukuda K, Chambers C et al: Bone morphogenetic protein 7 in dormancy and metastasis of prostate cancer stem-like cells in bone. J Exp Med 2011, 208(13): 2641-2655.
- Park M, Cho YJ, Kim B, Ko YJ, Jang Y, Moon YH, Hyun H, Lim W: RANKL immunisation inhibits prostate cancer metastasis by modulating EMT through a RANKL-dependent pathway. Sci Rep 2021, 11(1): 12186.
- Ono T, Hayashi M, Sasaki F, Nakashima T: RANKL biology: bone metabolism, the immune system, and beyond. Inflamm Regen 2020, 40: 2.
- Ren D, Dai Y, Yang Q, Zhang X: Wnt5a induces and maintains prostate cancer cells dormancy in bone. J Exp Med 2019, 216(2): 428-449.
- Cackowski FC, Heath EI: Prostate cancer dormancy and recurrence. Cancer Lett 2022, 524: 103-108.
- Lee GT, Kwon SJ, Kim J, Kwon YS, Lee N, Hong JH, Jamieson C, Kim WJ, Kim IY: WNT5A induces castration-resistant prostate cancer via CCL2 and tumour-infiltrating macrophages. Br J Cancer 2018, 118(5): 670-678.
- Lee GT, Kang DI, Ha YS, Jung YS, Chung J, Min K, Kim TH, Moon KH, Chung JM, Lee DH et al: Prostate cancer bone metastases acquire resistance to androgen deprivation via WNT5A-mediated BMP-6 induction. Br J Cancer 2014, 110(6): 1634-1644.
- Kaplan Z, Zielske SP, Ibrahim KG, Cackowski FC: Wnt and β-Catenin Signaling in the Bone Metastasis of Prostate Cancer. Life (Basel) 2021, 11(10): 1099.
- Pulianmackal AJ, Sun D, Yumoto K, Li Z, Chen YC, Patel MV, Wang Y, Yoon E, Pearson A, Yang Q et al: Monitoring Spontaneous Quiescence and Asynchronous Proliferation-Quiescence Decisions in Prostate Cancer Cells. Front Cell Dev Biol 2021, 9: 728663.
- Lawson MA, McDonald MM, Kovacic N, Hua Khoo W, Terry RL, Down J, Kaplan W, Paton-Hough J, Fellows C, Pettitt JA et al: Osteoclasts control reactivation of dormant myeloma cells by remodelling the endosteal niche. Nat Commun 2015, 6: 8983.
- Albrengues J, Shields MA: Neutrophil extracellular traps produced during inflammation awaken dormant cancer cells in mice. Science 2018, 361(6409): eaao4227.
- Liu Q, Li A, Tian Y, Wu JD, Liu Y, Li T, Chen Y, Han X, Wu K: The CXCL8-CXCR1/2 pathways in cancer. Cytokine Growth Factor Rev 2016, 31: 61-71.
- Danilucci TM, Santos PK, Pachane BC, Pisani GFD, Lino RLB, Casali BC, Altei WF, Selistre-de-Araujo HS: Recombinant RGD-disintegrin DisBa-01 blocks integrin α(v)β(3) and impairs VEGF signaling in endothelial cells. Cell Commun Signal 2019, 17(1): 27.
- Decker AM, Jung Y, Cackowski FC, Yumoto K, Wang J, Taichman RS: Sympathetic Signaling Reactivates Quiescent Disseminated Prostate Cancer Cells in the Bone Marrow. Mol Cancer Res 2017, 15(12): 1644-1655.
- Wu AC, He Y, Broomfield A, Paatan NJ: CD169(+) macrophages mediate pathological formation of woven bone in skeletal lesions of prostate cancer. J Pathol 2016, 239(2): 218-230.
- Strömvall K, Sundkvist K, Ljungberg B, Halin Bergström S, Bergh A: Reduced number of CD169(+) macrophages in pre-metastatic regional lymph nodes is associated with subsequent metastatic disease in an animal model and with poor outcome in prostate cancer patients. Prostate 2017, 77(15): 1468-1477.
- Lin SC, Lee YC, Yu G, Cheng CJ, Zhou X, Chu K, Murshed M, Le NT, Baseler L, Abe JI et al: Endothelial-to-Osteoblast Conversion Generates Osteoblastic Metastasis of Prostate Cancer. Dev Cell 2017, 41(5): 467-480.e463.
- Zhang Y, Liang J, Liu P, Wang Q, Liu L, Zhao H: The RANK/RANKL/OPG system and tumor bone metastasis: Potential mechanisms and therapeutic strategies. Front Endocrinol (Lausanne) 2022, 13: 1063815.
- Zhang B, Li Y, Wu Q, Xie L, Barwick B: Acetylation of KLF5 maintains EMT and tumorigenicity to cause chemoresistant bone metastasis in prostate cancer. Nat Commun 2021, 12(1): 1714.
- Sun CY, Mi YY, Ge SY, Hu QF, Xu K, Guo YJ, Tan YF, Zhang Y, Zhong F, Xia GW: Tumor- and Osteoblast-Derived Periostin in Prostate Cancer bone Metastases. Front Oncol 2021, 11: 795712.
- Saad F, Gleason DM, Murray R, Tchekmedyian S, Venner P, Lacombe L, Chin JL, Vinholes JJ, Goas JA, Chen B: A randomized, placebo-controlled trial of zoledronic acid in patients with hormone-refractory metastatic prostate carcinoma. J Natl Cancer Inst 2002, 94(19): 1458-1468.
- Finianos A, Aragon-Ching JB: Zoledronic acid for the treatment of prostate cancer. Expert Opin Pharmacother 2019, 20(6): 657-666.
- Fizazi K, Carducci M, Smith M, Damião R, Brown J, Karsh L, Milecki P, Shore N, Rader M, Wang H et al: Denosumab versus zoledronic acid for treatment of bone metastases in men with castration-resistant prostate cancer: a randomised, double-blind study. Lancet 2011, 377(9768): 813-822.
- Saad F, McKiernan J, Eastham J: Rationale for zoledronic acid therapy in men with hormone-sensitive prostate cancer with or without bone metastasis. Urol Oncol 2006, 24(1): 4-12.
- Saad F: Zoledronic acid significantly reduces pathologic fractures in patients with advanced-stage prostate cancer metastatic to bone. Clin Prostate Cancer 2002, 1(3): 145-152.
- Landgraf M, Lahr CA, Sanchez-Herrero A, Meinert C: Humanized bone facilitates prostate cancer metastasis and recapitulates therapeutic effects of zoledronic acid in vivo. Bone Res 2019, 7: 31.
- Landgraf M, Lahr CA, Sanchez-Herrero A, Meinert C, Shokoohmand A, Pollock PM, Hutmacher DW: Correction: Humanized bone facilitates prostate cancer metastasis and recapitulates therapeutic effects of Zoledronic acid in vivo. Bone Res 2020, 8: 17.
- Hegemann M, Bedke J, Stenzl A, Todenhöfer T: Denosumab treatment in the management of patients with advanced prostate cancer: clinical evidence and experience. Ther Adv Urol 2017, 9(3-4): 81-88.
- Sølling AS, Harsløf T: Discontinuation of denosumab in men with prostate cancer. Osteoporos Int 2023, 34(2): 291-297.
- Galvano A, Scaturro D, Badalamenti G, Incorvaia L, Rizzo S, Castellana L, Cusenza S, Cutaia S, Santini D, Guadagni F et al: Denosumab for bone health in prostate and breast cancer patients receiving endocrine therapy? A systematic review and a meta-analysis of randomized trials. J Bone Oncol 2019, 18: 100252.
- Paller CJ, Carducci MA, Philips GK: Management of bone metastases in refractory prostate cancer--role of denosumab. Clin Interv Aging 2012, 7: 363-372.
- Saad F, Sternberg CN, Mulders PFA, Niepel D, Tombal BF: The role of bisphosphonates or denosumab in light of the availability of new therapies for prostate cancer. Cancer Treat Rev 2018, 68: 25-37.
- Gunawan F, George E, Kotowicz M: Denosumab-induced hypocalcaemia in metastatic castrate-resistant prostate cancer. Endocrinol Diabetes Metab Case Rep 2019, 2019: 19-0063.
- Zhang N, Zhang ZK, Yu Y, Zhuo Z, Zhang G, Zhang BT: Pros and Cons of Denosumab Treatment for Osteoporosis and Implication for RANKL Aptamer Therapy. Front Cell Dev Biol 2020, 8: 325.
- Jakob T, Tesfamariam YM, Macherey S, Kuhr K, Adams A, Monsef I, Heidenreich A, Skoetz N: Bisphosphonates or RANK-ligand-inhibitors for men with prostate cancer and bone metastases: a network meta-analysis. Cochrane Database Syst Rev 2020, 12(12): Cd013020.
- van der Zande K, Oyen WJG: Radium-223 Treatment of Patients with Metastatic Castration Resistant Prostate Cancer: Biomarkers for Stratification and Response Evaluation. Cancers (Basel) 2021, 13(17): 4346.
- Bauckneht M, Capitanio S, Donegani MI, Zanardi E, Miceli A, Murialdo R, Raffa S, Tomasello L, Vitti M, Cavo A: Role of Baseline and Post-Therapy 18F-FDG PET in the Prognostic Stratification of Metastatic Castration-Resistant Prostate Cancer (mCRPC) Patients Treated with Radium-223. Cancers (Basel) 2019, 12(1): 31.
- Sindhu KK, Nehlsen AD, Stock RG: Radium-223 for Metastatic Castrate-Resistant Prostate Cancer. Pract Radiat Oncol 2022, 12(4): 312-316.
- Dandapani SV, Wong J, Twardowski P: Review of Radium-223 and Metastatic Castration-Sensitive Prostate Cancer. Cancer Biother Radiopharm 2020, 35(7): 490-496.
- Miranda J, Viñal D, Pinto Á: Radium 223 for the treatment of metastatic castration-resistant prostate cancer. Arch Esp Urol 2019, 72(5): 500-507.
- Parker C, Nilsson S, Heinrich D, Helle SI, O'Sullivan JM, Fosså SD, Chodacki A, Wiechno P, Logue J, Seke M et al: Alpha emitter radium-223 and survival in metastatic prostate cancer. N Engl J Med 2013, 369(3): 213-223.
- Gómez Rivas J, Carrion DM, Alvarez-Maestro M, Cathelineau X, Sanchez-Salas R, Di Lorenzo G, Di Maio M, Paul A, Martinez-Piñeiro L, Sartor O et al: Bone-targeted therapy in castration-resistant prostate cancer: where do we stand? Minerva Urol Nefrol 2019, 71(5): 445-456.
- Zeng X, Liu Q, Tan C, Wan X, Wang Y, Ma X: Alpha emitter radium-223 in patients with metastatic castration-resistant prostate cancer: A cost-utility analysis. Front Pharmacol 2022, 13: 1003483.
- Nilsson S: Alpha-emitter radium-223 in the management of solid tumors: current status and future directions. Am Soc Clin Oncol Educ Book 2014: e132-139.
- Sartor O, Sharma D: Radium and other alpha emitters in prostate cancer. Transl Androl Urol 2018, 7(3): 436-444.
- Hu Z, Tian Y, Li W, Ruan Y, Zeng F: The efficacy and safety of zoledronic acid and strontium-89 in treating non-small cell lung cancer: a systematic review and meta-analysis of randomized controlled trials. Support Care Cancer 2020, 28(7): 3291-3301.
- Baba K, Kaida H, Hattori C, Muraki K, Kugiyama T, Fujita H, Ishibashi M: Tumoricidal effect and pain relief after concurrent therapy by strontium-89 chloride and zoledronic acid for bone metastases. Hell J Nucl Med 2018, 21(1): 15-23.
- Furubayashi N, Negishi T, Ura S, Hirai Y, Nakamura M: Palliative effects and adverse events of strontium-89 for prostate cancer patients with bone metastasis. Mol Clin Oncol 2015, 3(1): 257-263.
- Altman GB, Lee CA: Strontium-89 for treatment of painful bone metastasis from prostate cancer. Oncol Nurs Forum 1996, 23(3): 523-527.
- Porter AT: Strontium-89 (Metastron) in the treatment of prostate cancer metastatic to bone. Eur Urol 1994, 26 Suppl 1: 20-25.
- Zhao WW, Xie P, Deng HF: [Strontium-89 for bone metastases from prostate cancer: an update]. Zhonghua Nan Ke Xue 2010, 16(3): 269-272.
- Murray I, Du Y: Systemic Radiotherapy of Bone Metastases With Radionuclides. Clin Oncol (R Coll Radiol) 2021, 33(2): 98-105.
- Fioramonti M, Santini D, Iuliani M, Ribelli G, Manca P, Papapietro N, Spiezia F, Vincenzi B, Denaro V, Russo A et al: Cabozantinib targets bone microenvironment modulating human osteoclast and osteoblast functions. Oncotarget 2017, 8(12): 20113-20121.
- Schöffski P, Gordon M, Smith DC, Kurzrock R, Daud A, Vogelzang NJ, Lee Y, Scheffold C, Shapiro GI: Phase II randomised discontinuation trial of cabozantinib in patients with advanced solid tumours. Eur J Cancer 2017, 86: 296-304.
- Smith DC, Daignault-Newton S, Grivas P, Reichert ZR, Hussain M, Cooney KA, Caram M, Alva A, Jacobson J, Yablon C et al: Efficacy and Effect of Cabozantinib on Bone Metastases in Treatment-naive Castration-resistant Prostate Cancer. Clin Genitourin Cancer 2020, 18(4): 332-339.e332.
- Paindelli C, Casarin S, Wang F, Diaz-Gomez L, Zhang J, Mikos AG, Logothetis CJ, Friedl P, Dondossola E: Enhancing (223)Ra Treatment Efficacy by Anti-β1 Integrin Targeting. J Nucl Med 2022, 63(7): 1039-1045.
- Hill EE, Kim JK: Integrin alpha V beta 3 targeted dendrimer-rapamycin conjugate reduces fibroblast-mediated prostate tumor progression and metastasis. J Cell Biochem 2018, 119(10): 8074-8083.
Annals of urologic oncology
p-ISSN: 2617-7765, e-ISSN: 2617-7773
Copyright © Ann Urol Oncol. This work is licensed under a Creative Commons Attribution-NonCommercial-No Derivatives 4.0 International (CC BY-NC-ND 4.0) License.