Review Article | Open Access
Role of Hyperbaric Oxygen Therapy in Prostate Tumor Microenvironment and Cancer Stem Cell Niche
Pranathi Konda1, Merrel Holley2, Vinoth Kumar Lakshmanan1
1Prostate Cancer Biomarker Laboratory, Faculty of Clinical Research, Sri Ramachandra Institute of Higher Education & Research, Porur, Chennai-600116, India.
2International Hyperbaric Medical Foundation, Morgan City, LA, USA.
Correspondence: Vinoth Kumar Lakshmanan (Prostate Cancer Biomarker Laboratory, Faculty of Clinical Research, Sri Ramachandra Institute of Higher Education & Research, Porur, Chennai-600116, India; Email: vinoth.lakshmanan@sriramachandra.edu.in).
Annals of Urologic Oncology 2024, 7(4): 185-194. https://doi.org/10.32948/auo.2024.12.01
Received: 15 Oct 2024 | Accepted: 03 Dec 2024 | Published online: 12 Dec 2024
Key words hyperbaric oxygen, hypoxia, tumor microenvironment, cancer stem cells, therapy
For HBOT to be efficacious, it can be administered through inhalation in the ambient environment, via an endotracheal tube within a monoplace chamber, or through masks, well fitting hoods, or endotracheal tubes within a larger multi-occupant chamber. The duration and barometric pressure of individual treatments may also varry, spanning from 45 minutes for carbon monoxide poisoning to nearly 5 hours for certain severe decompression disorders [12]. The decision regarding the use and qualification for HBOT was made by a collaborative team consisting of plastic surgeons, anesthesiologists, and intensive care specialists, in alignment with the guidelines set forth by the Undersea and Hyperbaric Medical Society and the criteria established by the European consensus conference on hyperbaric medicine [13]. Recent studies suggest that pre-surgical HBOT may reduce complications and it can also be combined with surgery, radiotherapy, chemotherapy, and photodynamic therapy [14, 15].
HBOT is extensively employed as a post-injury treatment [16]. HBOT chambers, which are hermetically sealed, elevate barometric pressure and provide >92% O for breathing. Treatments generally involve pressurizing the environment to 1.5-3.0 ₂ ATA for 60-120 minutes, usually once or multiple times daily [17, 18]. HBOT can be administered through inhalation in the ambient environment, endotracheal tubes in monoplace chambers, or masks and hoods in multi-occupant chambers [19]. HBOT accelerates tissue healing and enhances physiological aspects by increasing oxygen delivery to injured areas, enhancing the oxygen diffusion gradient, and improving oxygen saturation in blood plasma, which helps overcome compromised blood flow and supports cell growth and wound healing (Figure 1) [20-22]. Fortunately, the side effects of HBOT are typically mild [23]. The therapeutic impact of HBOT is attributed to at least five mechanisms: epigenetic regulation of gene expression, mechanical effects, bacteriostatic effects, hyperoxygenation for carbon monoxide and cyanide poisoning treatment, and restoration from hypoxia [24, 25].
HBOT modifies signalling pathways related to hypoxia and wound healing, notably affecting hypoxia inducible factor (HIF) and heme-oxygenase (HO) pathways [26]. It also influences apoptotic pathways, including the mitochondrial pathway, tumor suppressive endoplasmic reticulum stress (ERS), and autophagy due to decreased ratio of Bcl-2/Bax, increased level of p53, cleaved Caspase3, GRP78, CHOP, and LC3 in response to HBOT combined with melatonin on gastric cancer [27]. In severe brain disorders, HBOT reduces flammation, suppresses proinflammatory cytokines (IL-1β, IL-12, TNFα, IFNγ), and boosts the anti-inflammatory cytokine IL-10, indicating potential cytoprotective effects (Figure 1) [28]. HBOT can stimulate angiogenesis in various organs (Figure 1) [29]. HBOT has been extensively documented for its ability to improve Late Radiation Tissue Injury (LRTI) in various tissue types (Figure 1) [30]. HBOT is known to impede the advancement of genetically restricted autoimmune manifestations (Figure 1) [31]. While HBOT presents significant therapeutic potential, it is not without limitations, as discussed later in this paper.
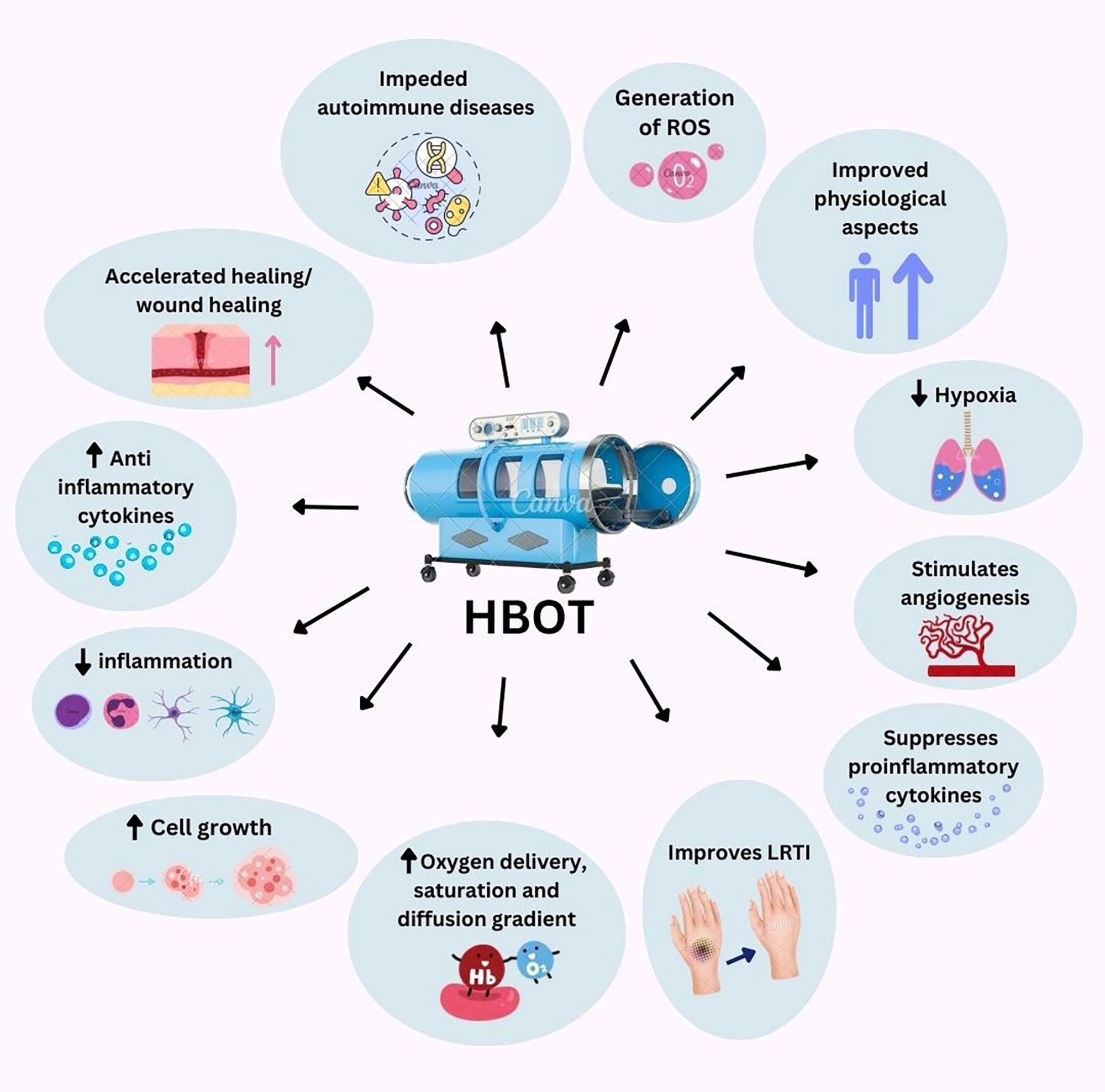
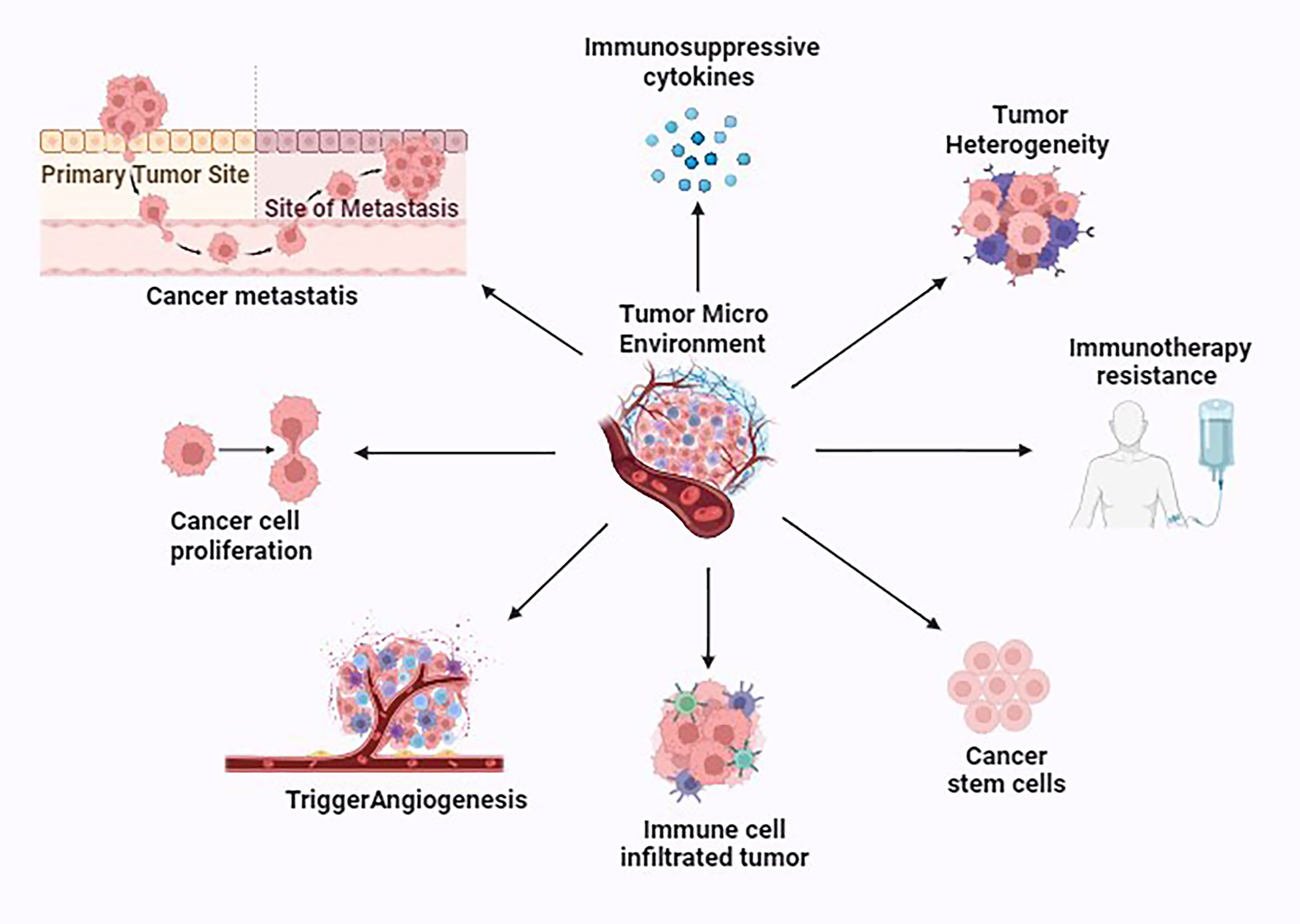
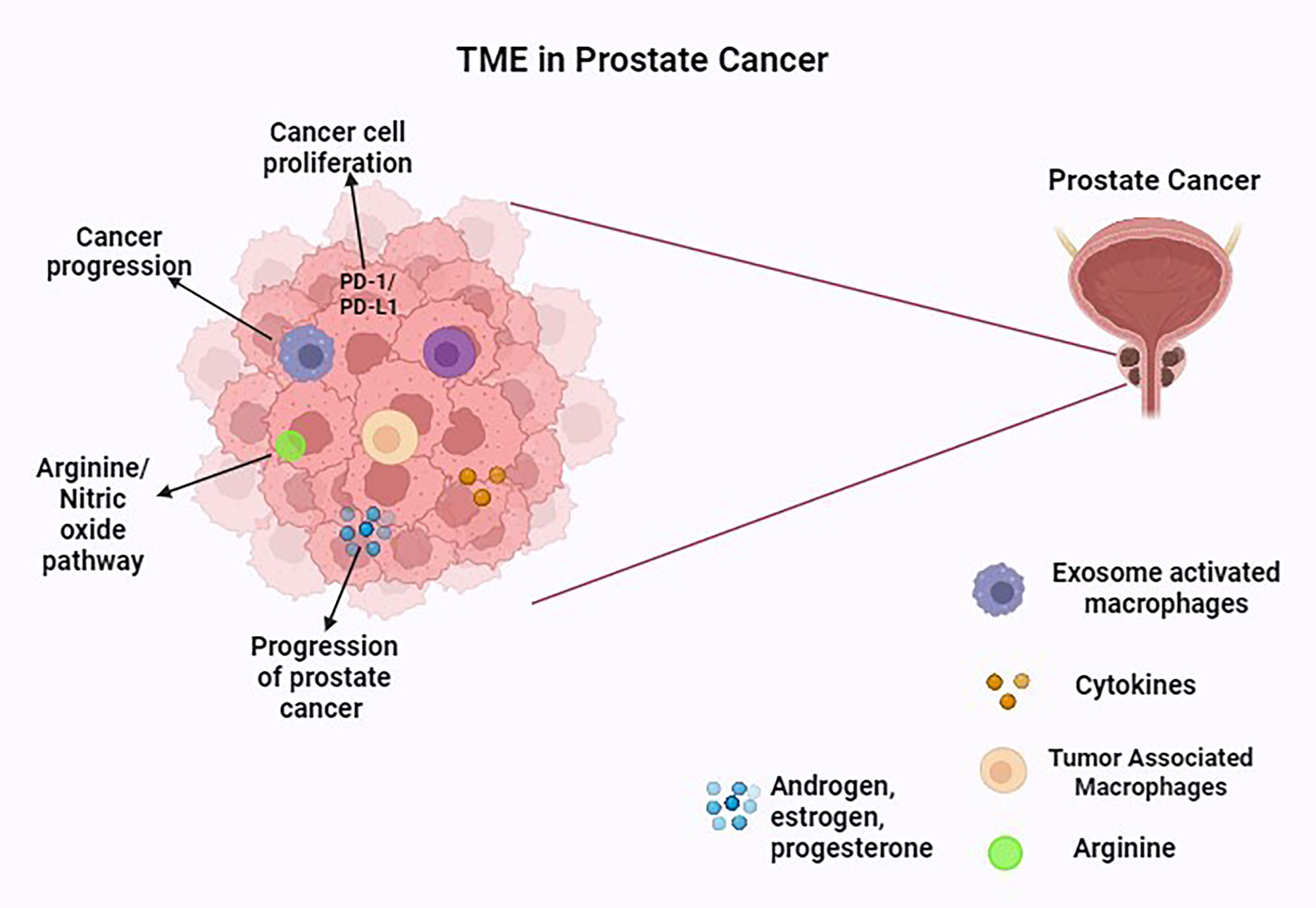
Usually, the TME is the one that serves as a pivotal role in preserving the stem-like characteristics of Cancer. In the case of prostate tumor, a distinct subpopulation of cells possessing stem cell-like traits, including the expression of stem cell markers CD44 and CD133, is recognized as cancer stem cells or cancer repopulating cells (CRCs). These cells possess the proliferative capacity to sustain the tumor mass and they also exhibit resistance to chemotherapy, enabling them to repopulate the tumor and instigate metastasis following cancer treatment (Figure 4) [75]. It is believed that the presence of rare intermediate cells, which display a combination of CK5, CK8/18, and prostate stem cell antigen (PSCA), signifies the progenitor or transit amplifying cell population [76]. Recent findings indicate that calcitonin (CT), a hormone that lowers the amount of calcium in the bloodstream when secreted by the thyroid gland induces the expression of stem cell markers and other traits associated with CRC in PCa cells and enhances the metastatic potential of PCa cells by triggering epithelial to-mesenchymal transition (EMT) (Figure 4) [77]. miRNAs are also found to play an important role in regulating the stemness of PCSCs, both through direct control of transcription factors and biomarkers associated with stemness, indirectly impacting the process of EMT [78]. Mutations affecting the BAZ2A-bromodomain or the use of chemical agents that disrupt the binding between BAZ2A-bromodomain and H3K14ac have a detrimental impact on prostate cancer stem cells. Additionally, the pharmacological inhibition of BAZ2A-BRD hinders the oncogenic transformation resulting from Pten loss in the case of prostate organoids [77]. For the forthcoming patient-specific PCa therapies, NF-kB pathways can be used which plays a potential role by eradicating PCSCs. Treatment with TNFα was also shown to successively eliminate PCSCs (Figure 4) [79].
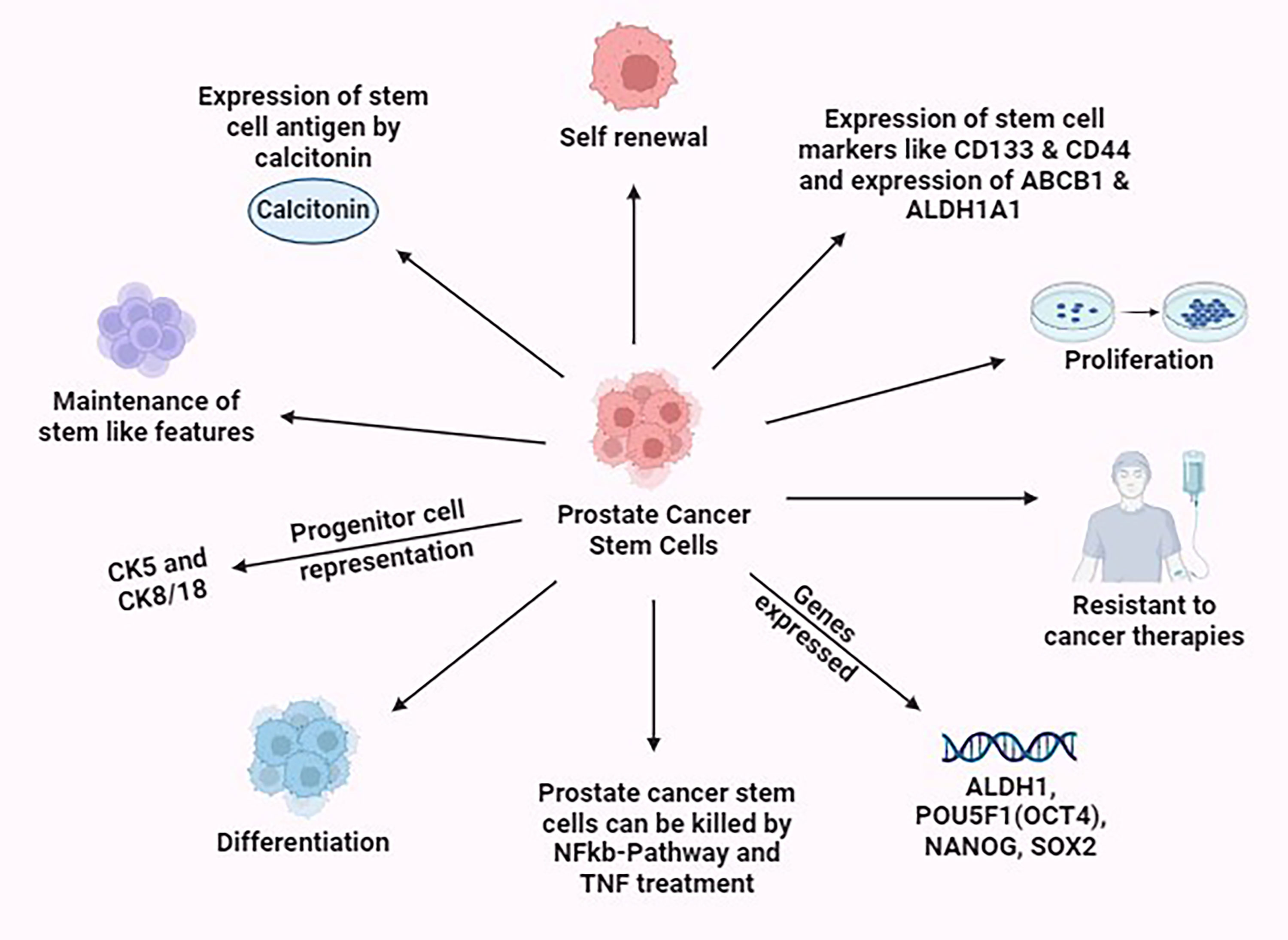
In addition to the previously mentioned limitations, logistical barriers can also pose challenges in the administration of HBOT. For instance, a qualitative study involving podiatrists from high-risk foot clinics near Sydney revealed that participants considered the evidence supporting HBOT for diabetic foot ulcers (DFU) to be extremely limited. Many studies were criticized for lacking scientific rigor, leading the podiatrists to recommend HBOT referrals only when requested by the patient [90].
Over the decades, PCSCs have been a focal point in cancer research due to their significant role in tumor initiation, progression, and resistance to conventional therapies [91]. These cells possess the unique ability to self-renew and differentiate, which makes them a critical target in the fight against prostate cancer. The resistance of PCSCs to conventional therapies is a major challenge, as these cells can survive treatment and lead to tumor relapse and metastasis. Therefore, developing therapies that specifically target PCSCs is crucial for improving treatment outcomes. By focusing on strategies that eliminate these resilient cells, we can potentially reduce the risk of recurrence and improve the overall efficacy of prostate cancer treatments in combination with HBOT. This approach holds promise for more effective and lasting cancer therapy, bringing us closer to better patient outcomes. In addition, nanotechnology platforms allow for precise control over the size and shape of nanomaterials, which can be optimized to target cancer cells more effectively. The combination of these nanomaterials with antibodies or nanobodies can further enhance their specificity and therapeutic impact for Prostate cancer [92] and offers exciting potential in targeting prostate cancer stem cells (PCSCs) in combination of HBOT whereby significant enhancement on therapeutic outcomes by specifically targeting PCSCs, thereby improving efficacy and reducing stemness features can be achieved and vital role of biomarkers [93] can be addressed for precised treatment.
None.
Ethical policy
Non applicable.
Availability of data and materials
No data was used for the research described in the article.
Author contributions
The study was directed by MH and VKL. PK searched academic literature, wrote the manuscript draft. MH and VKL supervised the review draft and approved the final manuscript submission.
Competing interests
MH is the current president of the International Hyperbaric Medical Foundation, a 501(c)3 nonprofit and holds multiple patents on hyperbaric technologies. The authors declare that they have no known competing financial interests or personal relationships that could have appeared to influence the work reported in this review.
Funding
This study was financially supported by the Department of Science and Technology (DST), SERB (Research Grant number CRG/2021/001369) to V-KL.
- Leach RM, Rees PJ, Wilmshurst P: Hyperbaric oxygen therapy. BMJ 1998, 317(7166): 1140-1143.
- Noyer CM, Brandt LJ: Hyperbaric oxygen therapy for perineal Crohn's disease. Am J Gastroenterol 1999, 94(2): 318-321.
- Marcinkowska AB, Mankowska ND, Kot J, Winklewski PJ: Impact of hyperbaric oxygen therapy on cognitive functions: a systematic review. Neuropsychol Rev 2022, 32(1): 99-126.
- Dauwe PB, Pulikkottil BJ, Lavery L, Stuzin JM, Rohrich RJ: Does hyperbaric oxygen therapy work in facilitating acute wound healing? Plast Reconstr Surg 2014, 133(2): 208e-215e.
- Cozene B, Sadanandan N, Gonzales-Portillo B, Saft M, Cho J, Park YJ, Borlongan CV: An Extra Breath of Fresh Air: Hyperbaric Oxygenation as a Stroke Therapeutic. Biomolecules 2020, 10(9): 1279.
- Villanueva E, Bennett MH, Wasiak J, Lehm JP: Hyperbaric oxygen therapy for thermal burns. Cochrane Database Syst Rev 2004, 2004(3): CD004727.
- Levett D, Bennett MH, Millar I: Adjunctive hyperbaric oxygen for necrotizing fasciitis. Cochrane Database Syst Rev 2015, 1(1): CD007937.
- Memar MY, Yekani M, Alizadeh N, Baghi HB: Hyperbaric oxygen therapy: antimicrobial mechanisms and clinical application for infections. Biomed Pharmacother 2019, 109: 440-447.
- Schottlender N, Gottfried I, Ashery U: Hyperbaric oxygen treatment: effects on mitochondrial function and oxidative stress. Biomolecules 2021, 11(12): 1827.
- Sethuraman KN, Smolin R, Henry S: Is there a place for hyperbaric oxygen therapy? Adv Surg 2022, 56(1): 169-204.
- Iyikesici MS: Feasibility study of metabolically supported chemotherapy with weekly carboplatin/paclitaxel combined with ketogenic diet, hyperthermia and hyperbaric oxygen therapy in metastatic non-small cell lung cancer. Int J Hyperthermia 2019, 36(1): 446-455.
- Tibbles PM, Edelsberg JS: Hyperbaric-oxygen therapy. N Engl J Med 1996, 334(25): 1642-1648.
- Mladenov A, Diehl K, Müller O, von Heymann C, Kopp S, Peitsch WK: Outcome of necrotizing fasciitis and Fournier's gangrene with and without hyperbaric oxygen therapy: a retrospective analysis over 10 years. World J Emerg Surg 2022, 17(1): 43.
- De Wolde SD, Hulskes RH, Weenink RP, Hollmann MW, Van Hulst RA: The effects of hyperbaric oxygenation on oxidative stress, inflammation and angiogenesis. Biomolecules 2021, 11(8): 1210.
- Xue T, Ding JS, Li B, Cao DM, Chen G: A narrative review of adjuvant therapy for glioma: hyperbaric oxygen therapy. Med Gas Res 2021, 11(4): 155-157.
- Lippert T, Borlongan CV: Prophylactic treatment of hyperbaric oxygen treatment mitigates inflammatory response via mitochondria transfer. CNS Neurosci Ther 2019, 25(8): 815-823.
- Bennett M, Best TM, Babul S, Taunton J, Lepawsky M: Hyperbaric oxygen therapy for delayed onset muscle soreness and closed soft tissue injury. Cochrane Database Syst Rev 2005, 2005(4): CD004713.
- Bennett MH, Trytko B, Jonker B: Hyperbaric oxygen therapy for the adjunctive treatment of traumatic brain injury. Cochrane Database Syst Rev 2004, (4): CD004609. Update in: Cochrane Database Syst Rev 2012, 12: CD004609.
- Tibbles PM, Edelsberg JS: Hyperbaric-oxygen therapy. N Engl J Med 1996, 334(25): 1642-1648.
- Fischer I, Barak B: Molecular and therapeutic aspects of hyperbaric oxygen therapy in neurological conditions. Biomolecules 2020, 10(9): 1247.
- Holland NJ, Bernstein JM, Hamilton JW: Hyperbaric oxygen therapy for Bell's palsy. Cochrane Database Syst Rev 2012, 2012(2): CD007288.
- Green A, Hossain T, Eckmann DM: Hyperbaric oxygen alters intracellular bioenergetics distribution in human dermal fibroblasts. Life Sci 2021, 278: 119616.
- Thom SR: Hyperbaric-oxygen therapy for acute carbon monoxide poisoning. N Engl J Med 2002, 347(14): 1105-1106.
- Löndahl M: Hyperbaric oxygen therapy as treatment of diabetic foot ulcers. Diabetes Metab Res Rev 2012, 28(Suppl 1): 78-84.
- Prime Scholars: Hypoxia as an epigenetic factor and hyperbaric oxygen therapy. Available online.
- Dulai PS, Gleeson MW, Taylor D, Holubar SD, Buckey JC, Siegel CA: Systematic review: The safety and efficacy of hyperbaric oxygen therapy for inflammatory bowel disease. Aliment Pharmacol Ther 2014, 39(11): 1266-1275.
- Wei X, Qi Y, Jia N, Zhou Q, Zhang S, Wang Y: Hyperbaric oxygen treatment sensitizes gastric cancer cells to melatonin-induced apoptosis through multiple pathways. J Cell Biochem 2018, 119(8): 6723-6731.
- Mensah-Kane P, Sumien N: The potential of hyperbaric oxygen as a therapy for neurodegenerative diseases. GeroScience 2022, 45(2): 747-756.
- Hadanny A, Lang E, Copel L, Meir O, Bechor Y, Fishlev G, Bergan J, Friedman M, Zisman A, Efrati S: Hyperbaric oxygen can induce angiogenesis and recover erectile function. Int J Impot Res 2018, 30(6): 292-299.
- Bennett MH, Feldmeier J, Hampson NB, Smee R, Milross C: Hyperbaric oxygen therapy for late radiation tissue injury. Cochrane Database Syst Rev 2016.
- Saito K, Tanaka Y, Ota T, Eto S, Yamashita U: Suppressive effect of hyperbaric oxygenation on immune responses of normal and autoimmune mice. Clin Exp Immunol 1991, 86(2): 322-327.
- Bui QC, Lieber M, Withers HR, Corson K, van Rijnsoever M, Elsaleh H: The efficacy of hyperbaric oxygen therapy in the treatment of radiation-induced late side effects. Int J Radiat Oncol Biol Phys 2004, 60(3): 871-878.
- Henk JM, Smith CW: Radiotherapy and hyperbaric oxygen in head and neck cancer. Interim report of second clinical trial. Lancet 1977, 2(8029): 104-105.
- Ogawa K, Ishiuchi S, Inoue O, Yoshii Y, Saito A, Watanabe T, Iraha S, Toita T, Kakinohana Y, Ariga T: Phase II trial of radiotherapy after hyperbaric oxygenation with multiagent chemotherapy (procarbazine, nimustine, and vincristine) for high-grade gliomas: long-term results. Int J Radiat Oncol Biol Phys 2012, 82(2): 732-738.
- Ogawa K, Yoshii Y, Inoue O, Toita T, Saito A, Kakinohana Y, Adachi G, Ishikawa Y, Kin S, Murayama S: Prospective trial of radiotherapy after hyperbaric oxygenation with chemotherapy for high-grade gliomas. Radiother Oncol 2003, 67(1): 63-67.
- Bennett MH, Feldmeier J, Smee R, Milross C: Hyperbaric oxygenation for tumour sensitisation to radiotherapy. Cochrane Database Syst Rev 2018, 4(4): CD005007.
- Selvendiran K, Kuppusamy ML, Ahmed S, Bratasz A, Meenakshisundaram G, Rivera BK, Khan M, Kuppusamy P: Oxygenation inhibits ovarian tumor growth by downregulating STAT3 and cyclin-D1 expressions. Cancer Biol Ther 2010, 10(4): 386-390.
- Hoogsteen IJ, Marres HA, van der Kogel AJ, Kaanders JH: The hypoxic tumour microenvironment, patient selection and hypoxia-modifying treatments. Clin Oncol (R Coll Radiol) 2007, 19(6): 385-396.
- Wu X, Zhu Y, Huang W, Li J, Zhang B, Li Z, Yang X: Hyperbaric oxygen potentiates Doxil antitumor efficacy by promoting tumor penetration and sensitizing cancer cells. Adv Sci (Weinh) 2018, 5(8): 1700859.
- Bi J, Witt E, Voltarelli VA, Feig VR, Venkatachalam V, Boyce H, McGovern M, Gutierrez WR, Rytlewski JD, Bowman KR, et al: Low-cost, high-pressure-synthesized oxygen-entrapping materials to improve treatment of solid tumors. Adv Sci (Weinh) 2023, 10(10): e2205995.
- Daruwalla J, Christophi C: Hyperbaric oxygen therapy for malignancy: a review. World J Surg 2006, 30(12): 2112-2131.
- Fenton BM, Lord EM, Paoni SF: Intravascular HBO₂ saturations, perfusion and hypoxia in spontaneous and transplanted tumor models. Int J Cancer 2001, 93(5): 693-698.
- Stępień K, Ostrowski RP, Matyja E: Hyperbaric oxygen as an adjunctive therapy in treatment of malignancies, including brain tumours. Med Oncol 2016, 33(9): 101.
- Moen I, Stuhr LE: Hyperbaric oxygen therapy and cancer--a review. Target Oncol. 2012, 7(4): 233-242.
- Zembrzuska K, Ostrowski RP, Matyja E: Hyperbaric oxygen increases glioma cell sensitivity to antitumor treatment with a novel isothiourea derivative in vitro. Oncol Rep 2019, 41(5): 2703-2716.
- Rojas A, Stewart FA, Smith KA, Soranson JA, Randhawa VS, Stratford MR, Denekamp J: Effect of anemia on tumor radiosensitivity under normo and hyperbaric conditions. Int J Radiat Oncol Biol Phys 1987, 13(11): 1681-1689.
- Shi T, Zhu J, Zhang X, Mao X: The Role of Hypoxia and Cancer Stem Cells in Development of Glioblastoma. Cancers (Basel) 2023, 15(9): 2613.
- Al-Waili NS, Butler GJ: A combination of radiotherapy, nitric oxide and a hyperoxygenation sensitizing protocol for brain malignant tumor treatment. Med Hypotheses 2007, 68(3): 528-537.
- Li J, Huang J, Ao Y, Li S, Miao Y, Yu Z, Zhu L, Lan X, Zhu Y, Zhang Y, et al: Synergizing upconversion nanophotosensitizers with hyperbaric oxygen to remodel the extracellular matrix for enhanced photodynamic cancer therapy. ACS Appl Mater Interfaces 2018, 10(27): 22985-22996.
- Wang X, Li S, Liu X, Wu X, Ye N, Yang X, Li Z: Boosting nanomedicine efficacy with hyperbaric oxygen therapy. Adv Exp Med Biol 2021, 1295: 77-95.
- Arneth B: Tumor microenvironment. Medicina (Kaunas) 2019, 56(1): 15.
- Butturini E, Carcereri de Prati A, Boriero D, Mariotto S: Tumor dormancy and interplay with hypoxic tumor microenvironment. Int J Mol Sci 2019, 20(17): 4305.
- Picoli CC, Gonçalves BÔP, Santos GSP, Rocha BGS, Costa AC, Resende RR, Birbrair A: Pericytes cross-talks within the tumor microenvironment. Biochim Biophys Acta Rev Cancer 2021, 1876(2): 188608.
- Pansy K, Uhl B, Krstic J, Szmyra M, Fechter K, Santiso A, Thüminger L, Greinix H, Kargl J, Prochazka K: Immune regulatory processes of the tumor microenvironment under malignant conditions. Int J Mol Sci 2021, 22(24): 13311.
- Pitt JM, Marabelle A, Eggermont A, Soria JC, Kroemer G, Zitvogel L: Targeting the tumor microenvironment: removing obstruction to anticancer immune responses and immunotherapy. Ann Oncol 2016, 27(8): 1482-1492.
- Hinshaw DC, Shevde LA: The tumor microenvironment innately modulates cancer progression. Cancer Res 2019, 79(18): 4557-4566.
- Sacco A, Battaglia AM, Botta C, Aversa I, Mancuso S, Costanzo F, Biamonte F: Iron metabolism in the tumor microenvironment—implications for anti-cancer immune response. Cells 2021, 10(2): 303.
- Osipov A, Saung MT, Zheng L, Murphy AG: Small molecule immunomodulation: the tumor microenvironment and overcoming immune escape. J Immunother Cancer 2019, 7(1): 224.
- Ngambenjawong C, Gustafson HH, Pun SH: Progress in tumor-associated macrophage (TAM)-targeted therapeutics. Adv Drug Deliv Rev 2017, 114: 206-221.
- Kise K, Kinugasa-Katayama Y, Takakura N: Tumor microenvironment for cancer stem cells. Adv Drug Deliv Rev 2016, 99(Pt B): 197-205.
- Jiang Y, Wells A, Sylakowski K, Clark AM, Ma B: Adult stem cell functioning in the tumor micro-environment. Int J Mol Sci 2019, 20(10): 2566.
- Schaue D, McBride WH: Opportunities and challenges of radiotherapy for treating cancer. Nat Rev Clin Oncol 2015, 12(9): 527-540.
- Mazzu YZ, Liao Y, Nandakumar S, Sjöström M, Jehane LE, Ghale R, Govindarajan B, Gerke TA, Lee GM, Luo JH, et al: Dynamic expression of SNAI2 in prostate cancer predicts tumor progression and drug sensitivity. Mol Oncol 2022, 16(13): 2451-2469.
- Matos A, Carvalho M, Bicho M, Ribeiro R: Arginine and arginases modulate metabolism, tumor microenvironment, and prostate cancer progression. Nutrients 2021, 13(12): 4503.
- Thienger P, Rubin MA: Prostate cancer hijacks the microenvironment. Nat Cell Biol 2021, 23(1): 3-5.
- Vitkin N, Nersesian S, Siemens DR, Koti M: The tumor immune contexture of prostate cancer. Front Immunol 2019, 10: 603.
- Baig MS, Roy A, Rajpoot S, Liu D, Savai R, Banerjee S, Kawada M, Faisal SM, Saluja R, Saqib U, et al: Tumor-derived exosomes in the regulation of macrophage polarization. Inflamm Res 2020, 69(5): 435-451.
- Palicelli A, Croci S, Bisagni A, Zanetti E, De Biase D, Melli B, Sanguedolce F, Ragazzi M, Zanelli M, Chaux A, et al: What do we have to know about PD-L1 expression in prostate cancer? A systematic literature review. Part 3: PD-L1, intracellular signaling pathways and tumor microenvironment. Int J Mol Sci 2021, 22(22): 12330.
- Boibessot C, Toren P: Sex steroids in the tumor microenvironment and prostate cancer progression. Endocr Relat Cancer 2018, 25(3): R179-R196.
- Cioni B, Zwart W, Bergman AM: Androgen receptor moonlighting in the prostate cancer microenvironment. Endocr Relat Cancer 2018, 25(6): R331-R349.
- Liu X, Li WJ, Puzanov I, Goodrich DW, Chatta G, Tang DG: Prostate cancer as a dedifferentiated organ: androgen receptor, cancer stem cells, and cancer stemness. Essays Biochem 2022, 66(4): 291-303.
- Mayer MJ, Klotz LH, Venkateswaran V: Metformin and prostate cancer stem cells: a novel therapeutic target. Prostate Cancer Prostatic Dis 2015, 18(4): 303-309.
- Verma S, Shankar E, Kalayci FNC, Mukunda A, Alassfar M, Singh V, Chan ER, MacLennan GT, Gupta S: Androgen deprivation induces transcriptional reprogramming in prostate cancer cells to develop stem cell-like characteristics. Int J Mol Sci 2020, 21(24): 9568.
- Sánchez BG, Bort A, Vara-Ciruelos D, Díaz-Laviada I: Androgen deprivation induces reprogramming of prostate cancer cells to stem-like cells. Cells 2020, 9(6): 1441.
- Aldahish A, Kale A, Aljameeli A, Shah GV: Calcitonin induces stem cell-like phenotype in prostate cancer cells. Endocr Relat Cancer 2019, 26(11): 815-828.
- Hu WY, Shi GB, Hu DP, Nelles JL, Prins GS: Actions of estrogens and endocrine disrupting chemicals on human prostate stem/progenitor cells and prostate cancer risk. Mol Cell Endocrinol 2012, 354(1-2): 63-73.
- Peña-Hernández R, Aprigliano R, Frommel SC, Pietrzak K, Steiger S, Roganowicz M, Lerra L, Bizzarro J, Santoro R: BAZ2A-mediated repression via H3K14ac-marked enhancers promotes prostate cancer stem cells. EMBO Rep 2021, 22(11): e53014.
- Coradduzza D, Cruciani S, Arru C, Garroni G, Pashchenko A, Jedea M, Zappavigna S, Caraglia M, Amler E, Carru C: Role of miRNA-145, 148, and 185 and stem cells in prostate cancer. Int J Mol Sci 2022, 23(3): 1626.
- Witte KE, Pfitzenmaier J, Storm J, Lütkemeyer M, Wimmer C, Schulten W, Czaniera N, Geisler M, Förster C, Wilkens L: Analysis of several pathways for efficient killing of prostate cancer stem cells: a central role of NF-κB RELA. Int J Mol Sci 2021, 22(16): 8901.
- Chong KT, Hampson NB, Bostwick DG, Vessella RL, Corman JM: Hyperbaric oxygen does not accelerate latent in vivo prostate cancer: implications for the treatment of radiation-induced haemorrhagic cystitis. BJU Int 2004, 94(9): 1275-1278.
- Lu QZ, Li X, Ouyang J, Li JQ, Chen G: Further application of hyperbaric oxygen in prostate cancer. Med Gas Res 2019, 8(4): 167-171.
- Tang H, Zhang ZY, Ge JP, Zhou WQ, Gao JP: Effects of hyperbaric oxygen on tumor growth in the mouse model of LNCaP prostate cancer cell line. Zhonghua Nan Ke Xue 2009, 15(8): 713-716.
- Nakada T, Nakada H, Yoshida Y, Nakashima Y, Banya Y, Fujihira T, Karasawa K: Hyperbaric oxygen therapy for radiation cystitis in patients with prostate cancer: a long-term follow-up study. Urol Int 2012, 89(2): 208-214.
- Kalns JE, Piepmeier EH: Exposure to hyperbaric oxygen induces cell cycle perturbation in prostate cancer cells. In Vitro Cell Dev Biol Anim 1999, 35(2): 98-101.
- Re L: The Molecular Key to Understanding the Medical Ozone Action. Int J Mol Sci 2024, 25(11): 6148.
- Bennett MH, French C, Schnabel A, Wasiak J, Kranke P: Normobaric and hyperbaric oxygen therapy for migraine and cluster headache. Cochrane Database Syst Rev 2008, 16(3): CD005219.
- Gawdi R, Cooper JS: Hyperbaric Contraindications. 2023 Apr 8. In: StatPearls [Internet]. Treasure Island (FL): StatPearls Publishing; 2024.
- Alpuim Costa D, Modas Daniel P, Vieira Branco J: The Role of Hyperbaric Oxygen Therapy in Pneumatosis Cystoides Intestinalis-A Scoping Review. Front Med (Lausanne). 2021, 8: 601872.
- Schiavo S, Brenna CTA, Albertini L, Djaiani G, Marinov A, Katznelson R: Safety of hyperbaric oxygen therapy in patients with heart failure: A retrospective cohort study. PLoS One 2024, 19(2): e0293484.
- Henshaw FR, Brennan L, MacMillan F: Perceptions of hyperbaric oxygen therapy among podiatrists practicing in high-risk foot clinics. Int Wound J 2018, 15(3): 375-382.
- Ramesh S, Selvakumar P, Ameer MY, Lian S, Abdullah Alzarooni AIM, Ojha S, Mishra A, Tiwari A, Kaushik A, Jung YD: State-of-the-art therapeutic strategies for targeting cancer stem cells in prostate cancer. Front Oncol 2023, 13: 1059441.
- Rather GA, Selvakumar P, Srinivas KS, Natarajan K, Kaushik A, Rajan P, Lee SR, Sing WL, Alkhamees M, Lian S: Facile synthesis of elastin nanogels encapsulated decursin for castrated resistance prostate cancer therapy. Sci Rep 2024, 14(1): 15095.
- Kumar Am S, Rajan P, Alkhamees M, Holley M, Lakshmanan VK: Prostate cancer theragnostics biomarkers: An update. Investig Clin Urol 2024, 65(6): 527-539.
Annals of urologic oncology
p-ISSN: 2617-7765, e-ISSN: 2617-7773
Copyright © Ann Urol Oncol. This work is licensed under a Creative Commons Attribution-NonCommercial-No Derivatives 4.0 International (CC BY-NC-ND 4.0) License.