Review Article | Open Access
Regulatory Role of Autophagy and Glucose Metabolic Reprogramming in the Malignant Progression of Bladder Cancer: A Review
Wei Sun1, Junxiong Peng1, Sen Pan1, Chuanlin Wang1, Mengjuan Yuan11Department of Urology, Chongqing University FuLing Hospital, School of Medicine, Chongqing University, Chongqing 408000, PR. China.
Correspondence: Mengjuan Yuan (Department of Urology, Chongqing University FuLing Hospital, School of Medicine, Chongqing University, NO. 2 Gao Suntang Road, Fuling District, Chongqing 408000, PR. China; Email: 584155062@qq.com).
Annals of Urologic Oncology 2024, 7(1): 10-17. https://doi.org/10.32948/auo.2024.02.10
Received: 19 Jan 2024 | Accepted: 10 Feb 2024 | Published online: 15 Feb 2024
Key words bladder cancer, autophagy, glycolysis, metabolic reprogramming, malignant progression
Cancer has many characteristic changes, one of the important characteristics is the change of energy metabolism, which is especially conducive to the formation of proliferation, invasion and metastasis [19, 20]. As the main energy supplier of cells, glucose is normally ingested by cells and eventually produces a large amount of adenosine triphosphate (ATP) in mitochondria through aerobic metabolism for energy supply. However, malignantr cells not only have the characteristics of rapid proliferation, but also have the characteristics of rapid metabolism. And often in low oxygen, starvation and other TME, so that it needs a faster way to metabolize sugar to produce the required energy and raw materials, that is, the aerobic glycolysis [21-23]. The aerobic glycolytic transformation of sugars in tumor cells was first proposed by Warburg [24], also known as the Warburg effect, which refers to the rapid production of ATP by high-speed glycolysis in cancer cells even when oxygen is sufficient, and the oxidative metabolism of mitochondria is no longer coupled. Most of the pyruvate produced is catalyzed by lactate dehydrogenase and converted into lactic acid in the cytoplasmic matrix. At first, Warburg believed that the occurrence of aerobic glycolysis was due to the damage of mitochondria, but later studies found that cancer cells still showed Warburg effect even when mitochondria were intact, and the reprogramming of glucose metabolism was closely related to the changes of mitochondrial DNA, oncogenes and tumor suppressor factors [25, 26]. Therefore, it is reasonable to suspect that the reprogramming of glucose metabolism in tumor cells may be involved in the process of tumorigenesis and development. In recent years, researchers have repeatedly found that there is abnormal glucose metabolic reprogramming in cancer cells of patients with bladder cancer, and the changes in its metabolic pattern may play a key role in the progressive growth of bladder cancer. Figure 1 shows that the relationship between autophagy and metabolism.
Development of drug resistance in cancer
The abnormal increase of glycolysis in bladder cancer cells is mainly attributed to the overexpression of glycolytic-related genes and the abnormal production or function of key glycolytic enzymes [23], which may promote the enhancement of drug resistance in cancer cells. Pyruvate dehydrogenase kinase (pyruvate dehydrogenasekinase, PDK) family regulation of pyruvate dehydrogenase activity, it can through the pyruvate metabolism of transferred to the cytoplasm from mitochondria, promote the formation of the aerobic glycolysis. It has been confirmed in other tumors that inhibiting PDK can effectively reverse tumor cells' resistance to chemotherapy drugs [27]. Woolbright et al [28]. found that the expression of PDK4 was significantly increased in highly malignant bladder cancer cells. After using PDK4 competitive inhibitor DCA and siRNA knockdown related gene expression, growth of tumor cells is inhibited in G0 ~ G1 phase, and the combination of cisplatin with bladder cancer chemotherapy can effectively increase the injury-killing effect of the drug on tumor cells. The authors believe that PDK4 can improve the rapid production of ATP and the resistance to apoptosis induced by mitochondrial depolarization induced by cisplatin by enhancing aerobic glycolysis, and then produce drug resistance to chemotherapy drugs [29, 30]. In addition, M-type propionate kinase M (PKM), as a subtype of pyruvate kinase M, regulates the conversion of phosphoenolpyruvate to pyruvate, and is also one of the rate-limiting enzymes of glycolysis [31]. Wang et al. [32] found that compared with normal bladder tissues, the expression of PKM2 subtype was much higher than that of PKM1, regardless of the low level of NMIBC or high level of MIBC, and reducing its abnormal expression could effectively inhibit the proliferation and drug resistance of cancer cells, and increase the killing effect of cisplatin on tumor cells. Moreover, in the experiment of drug resistance induction on three bladder cancer cells, it was found that with the increasing of cisplatin concentration, the drug resistance of cells was positively correlated with the detected PKM2 expression level. Although additional evidence shows that Warburg effect is related to the formation of drug resistance in bladder cancer, the specific mechanism is still unclear. It may be related to increased glucose intake and metabolic abnormalities, or it may affect other metabolic ways of tumor cells, including lipid metabolism and amino acid synthesis. Experiments in bladder cancer and other cancers have confirmed that reprogramming to inhibit the glucose metabolism of cancer cells can effectively reverse the drug resistance of cancer and increase the killing effect of drugs on tumors [33]. A large number of studies have proved that bladder cancer cells affect programmed apoptosis through changes in the expression level of key glycolytic enzymes and changes in mitochondrial metabolism, and thus develop drug resistance, which is a mainstream research direction. However, the expression of key enzymes or post-transcriptional modification may be caused by the increase or decrease of transcription factors or modification enzymes, or by changes in DNA itself. Both require extensive and in-depth research.
Role of metabolic reprogramming in malignancy of bladder cancer
Cancer cell lines with higher malignancy also showed higher glycolytic transformation. The study found that in breast cancer, the non-aggressive breast cancer cell lines consumed less glucose than the aggressive lines, despite being cultured in vitro under adequate oxygen, suggesting that the more malignant tumor cells may have more metabolic changes in glycolysis [21]. Similar experiments have been carried out in breast cancer and have led to further investigation. Conde et al. [34] conducted metabolic uptake and consumption of MIBC cell line TCCSUP and NMIBC cell line RT4 respectively, and found that although there was no significant difference in glucose transporter 3 (GLUT3) and sugar consumption between the two cell lines, compared the expression levels of related proteins. The GLUT1 and PFK protein expressions of TCCSUP cells with higher malignant degree were significantly increased, and the consumption of pyruvate and the production of lactic acid and alanine were also higher than those of RT4 cells. How these changes occur, and how they affect bladder cancer cell invasion and metastasis, may require further investigation.
In summary, although the Warburg effect was discovered and its impact on cancer was proposed nearly a hundred years ago, and its research has never stopped, the mechanism of mitochondrial function decline or inhibition related to the metabolic reprogramming process of glycolysis has not been fully understood. With the application of glucose analogue tracer technology, fluorodeoxyglucose-positron tomography, which further confirmed that both primary and metastatic sites showed higher glucose uptake rates in tumor patients with higher malignancies, the exploration of glucose metabolic reprogramming in cancer is still of great significance. Among a variety of cancer cells, bladder cancer cells, especially those with a higher degree of malignancy, have a higher degree of aerobic glycolysis, suggesting that bladder cancer may affect the progressive changes in invasion, proliferation, drug resistance formation and other processes. Exploring and blocking the upstream mechanism of its metabolic transformation can prevent the progression of its evil behavior to a certain extent. This provides a very promising new approach for bladder cancer and even pan-cancer treatment.
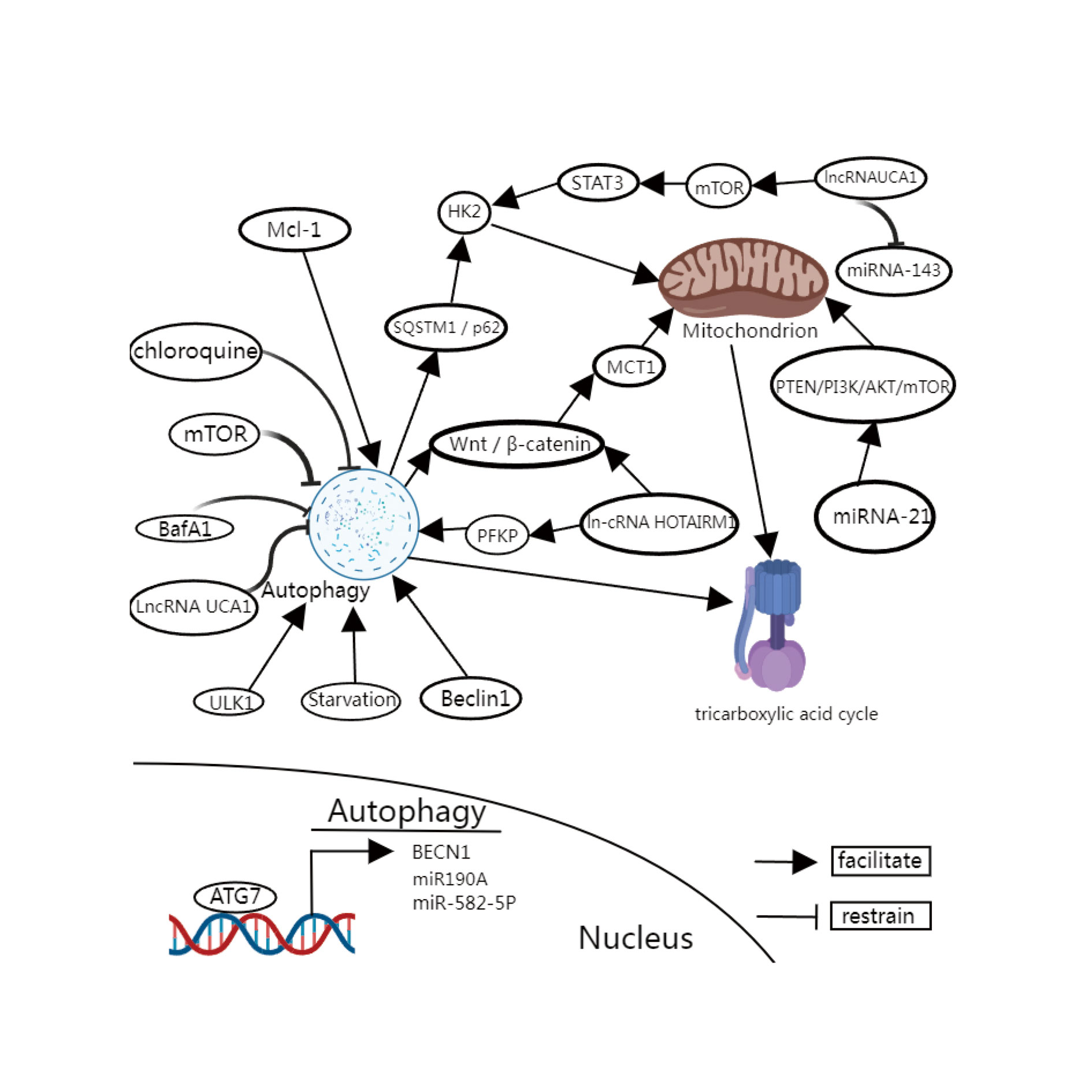
First proposed by Deduve et al in 1966 [35], autophagy is a conserved physiological catabolic process evolved in eukaryotic cells and is usually maintained at a basal level in somatic cells [36]. According to the different ways of transporting substances to lysosomes, autophagy can be divided into macroautophagy, microautophagy and chaperon-mediated autophagy. As the focus of research, macroautophagy plays a more important role. In this review, macroautophagy is mainly discussed as the main form of autophagy. Autophagy plays a double-edged role in the occurrence and development of cancer cells. When under stress, such as hypoxia, starvation, irradiation or chemotherapy, cells can up-regulate the autophagy level, wrap the aging organelles or proteins to form autophagy vesicles, and then combine with lysosomes to form autophagy - lysosome complex for further degradation. Thus prevent the accumulation of damaged proteins and organelles to maintain the homeostasis of the internal environment and play an anti-tumor role; But at the same time, the products of decomposition can also temporarily supply cells with energy, helping to cope with stimuli and maintain cell survival [37, 38]. Since the biological process of autophagy was proposed, more and more experiments have confirmed that autophagy may play a catalytic role in the advanced progression of bladder cancer and may cause key changes in the increased invasive ability of bladder cancer and the formation of drug resistance.
Autophagy related gene (ATG) and the malignant progression of bladder cancer
More and more studies have confirmed that the upregulation of ATG may be an important part of the progressive changes of bladder cancer. A series of proteins encoded by the ATG family participate in many processes related to autophagy, among which ATG7 is an essential autophagy factor, encoding E1 enzymes required for the lipidization of the ubiquitin-like egg white LC3 family and autophagosome formation, which has been explored in many studies [39]. Zhu et al. [40] compared invasive bladder cancer cells, clinical bladder cancer tissues and nude mouse allogeneic tumor tissues with normal urothelial cells and found that abnormally high expression of ATG7 was significantly correlated with increased tumor invasion ability, and further explored its mechanism. After screening the binding mirnas at the 3 'end of ATG7 mRNA, they found that miR190A can increase the stability of ATG7 mRNA by directly binding to the non-coding region of the 3' end of ATG7 mRNA, and then lead to the increase of ATG7 protein expression and the enhancement of autophagy level. After further investigation, it was found that ATG7 may enhance the ability of cysteal cancer invasion by increasing the level of autophagy. The mechanism is that ATG7 overexpression increases the autophagy digestion of HNRNPD protein in bladder cancer cells. RNA-binding protein immunoassay confirmed that HNRNPD protein could bind ARHGDIA mRNA to damage its translation, and the degradation of HNRNPD egg white increased the stability of ARHG-DIA mRNA. Thus, AR-HGDIA downstream of ATG7 is overexpressed, resulting in increased aggressiveness of bladder cancer cells. In this report, both in vivo and in vivo experiments confirmed that the increase of MIBC invading potential is a downstream event caused by the increase of autophagy level of ATG7, proving that autophagy promotes the progression of bladder cancer. Wu et al. [41] compared the cancer and adjacent tissues of patients with bladder cancer and found the high surface level of ln-cRNA UCA1 in urodermal epidermal embryonic antigen-1 (LN-CRNA UCA1). lncRNA UCA1 can prevent its binding to ATG7 by binding to miR-582-5p, thus increasing the expression of ATG7 and upregulating the level of autophagy. Moreover, lncRNA UCA1 can effectively inhibit autophagy of bladder cancer cells by knockout by shRNA thus inhibiting the proliferation, invasion and drug resistance of cancer cells. Not only ATG7, but also other members of the ATG family are closely related to the malignant progression of bladder cancer. Most studies have shown that synergistic changes of autophagy related genes and other regulatory factors may promote the progression of cancer cells. Regulation of related proteins, such as lncRNA or microRNA as ceRNAs, affects protein function or changes in protein modification, which may play an important role in cancer progression. At present, there are still few studies on ATG, and a large number of studies are still needed to confirm it in the future.
Autophagy and drug resistance in bladder cancer
Autophagy can be used as a protective response of cancer cells. In response to drugs, autophagy can be upregulated to resist apoptosis caused by drugs to escape the killing effect, thus inducing cancer cells to acquire drug resistance. Studies have shown that MIBC patients, especially those with T2 grade and above, receiving NAC can effectively degrade the tumor, which is beneficial to the surgery and prognosis of patients. The commonly used NAC regimen is mainly based on platinum-based drugs, such as the earliest MAVC (Methotrexate + vincristine + adriamycin + cisplatin) regimen. At present, the GC (gemcitabine + cisplatin) regimen and the GCarbo regimen proposed to replace cisplatin with carboplatin for drug intolerance are commonly used. However, a considerable number of patients are still resistant to chemotherapy drugs, which not only makes patients unable to benefit from the treatment, delays the treatment time, and brings the harm of adverse drug reactions to patients [42]. The mechanism of chemotherapy resistance is complex, and autophagy may be involved in its formation. Lin et al. [43] explored the drug sensitivity of high-grade bladder cancer cells to cisplatin, and found that cisplatin can cause BECN1 gene overexpression in bladder cancer cells, and through the influence of ATG7/12, autophagy level is increased, and autophagy can alleviate or prevent cisplatin induced apoptosis of bladder cancer cells to a certain extent. However, autophagy inhibitors chloroquine or BafA1 and BECN1 inhibition by shRNA inhibited autophagy increased apoptosis, suggesting that autophagy may be involved in cisplatin resistance formation. Jimenez-Guerrero et al. [44] found in their research on drug resistance of the anti-angiogenic drug paclitaxel in uroepithelial carcinoma that the combination of Mcl-1 inhibitor and paclitaxel could induce apoptosis of bladder cancer cells that were originally resistant to paclitaxel. The mechanism may be that the inhibitor inhibits autophagy of drug-resistant cells by increasing the breakdown of Beclin1. As well as inhibiting the ubiquitination of Mcl-1, thereby blocking the escape of S/G2 phase cells from mitotic slip and helping to overcome the resistance of resistant cells to paclitaxel.
In summary, autophagy, as a mechanism of cellular reactivity, may influence the progression of cancer cells through different signaling pathways in the process of cell cycle regulation or metabolic regulation during the advanced progression of bladder cancer, especially in its metastasis and the formation of drug resistance. In the process of chemotherapy targeting mitochondria, abnormal autophagy levels may act as an adaptive change of metabolism, leading to drug resistance of cancer cells and promoting the malignant transformation of bladder cancer [45-47].
Although abnormal proliferative ability and TME nutrient deficiency and harsh microenvironment seem to be contradictory in the process of cancer progression, the two do exist simultaneously. The possible explanation is to maximize the supply of substances related to the growth and progression of cancer cells by increasing the reuse of metabolites, as well as by degrading substances unrelated to growth or damaged organelles, and changing the metabolic pattern and structure of cells [48, 49]. In theory, metabolic reprogramming and autophagy can interact to supply most nutrients needed by cells. Autophagy plays an important role in the metabolic remodeling of cancer cells, and the core of the association is the tricarboxylic acid cycle. Many experiments have explored the metabolic reprogramming of cancer cells, including glucose metabolism, lipid metabolism and amino acid metabolism, and found that there is a close relationship with the progression of cancer, and autophagy plays a crucial role in it [50-53]. Strochecker et al. [53] found that knockdown of autophagy related gene ATG7 can affect the oxygen consumption rate of lung cancer cells and the growth of cancer cells by blocking autophagy, and adding exogenous glutamine to knockdown cells can restore the proliferation ability of cells. Therefore, it is believed that lung cancer cells can affect the reprogramming of glutamine metabolism through autophagy, which in turn affects the progressive growth of cancer cells. Ma et al. [54] found that abnormally high expression of lncRNA HAGLROS in bile duct cancer cells was significantly correlated with cancer growth and malignant progression. After the elimination of lncRNA HAGL-ROS, mTOR pathway could be inhibited to promote autophagy, thereby improving the lipid metabolic reprogramming of cancer cells, thus blocking the malignant process of cancer cells. Therefore, autophagy may play an upstream role in regulating the metabolic reprogramming of cancer cells, and thus affect the change of biological behavior of cancer cells.
Autophagy regulation of glucose metabolic reprogramming in cancer
There are many characteristics of cancer cells, one of which is the transformation of glucose metabolism from oxidative phosphorylation to glycolysis, which is also known as the Warburg effect. The influence of autophagy in this phenomenon and the progression of cancer cellularity have been well studied. TME plays multiple roles. When cells are in a nutritionally adequate environment, rapamycin complex 1 (MTORC1) promotes cell growth and inhibits autophagy by inhibiting ULK1, while AMPK is activated when cells are inadequately supplied with energy. The inhibition of MTORC1 and activation of ULK1 regulated the occurrence of autophagy, suggesting the interaction between glucose metabolism and autophagy [55, 56]. Other studies have shown that miRNA-21 in bladder cancer cells can enhance aerobic glycolysis of cancer cells through the PTEN/PI3K/AKT/mTOR signaling pathway, and lentiviruses targeting this target can effectively inhibit this effect [57]. lncRNAUCA1 is one of the earliest lncrnas found to be overexpressed in bladder cancer [58]. Li et al. [59] found that lncRNAUCA1 can exert hexokinase (HK2) activity and thus trigger the Warburg effect of cells. The specific mechanism is that ln-cRNA UCA1 activates STAT3 and inhibits miRNA-143 through mTOR. Although there have been few studies in bladder cancer, the regulation of HK2 activity plays a role in the switch of autophagy regulation of glycolysis in liver cancer. Lys63 ubiquitination of HK2 in liver cancer cells is mediated by ubiquitin ligase TRAF6. Autophagy can recognize and selectively degrade ubiquitin HK2 by the SQSTM1 / p62 receptor. Thus, aerobic glycolysis and proliferation of liver cancer cells are inhibited [60]. In addition, monocarboxylic acid transporter, as an important protein to transport lactic acid and remove hydrogen ions, also plays an important role in the regulation of cancer metabolism and autophagy [61]. Overexpression of MCT1 is significantly correlated with cancer progression and prognosis in patients with bladder cancer. Knockdown of MCT1 can significantly reduce the activity of key enzymes in the glycolytic pathway, reduce the production of lactic acid, and delay the malignant progression of bladder cancer cells, such as proliferation, invasion, metastasis and epithelial mesenchymal transformation, confirming that the change of Warburg effect in bladder cancer cells is positively correlated with malignant progression [62]. Starvation induces the enhancement of autophagy level in hepatocellular carcinoma cells, which can positively regulate the overexpression of MCT1 through Wnt / β-catenin signaling pathway, and promote the occurrence of aerobic glycolysis and the enhancement of invasion ability of cancer cells [63]. In addition, knockdown of ln-cRNA HOTAIRM1 in acute myeloid leukemia can also effectively inhibit the activity of P-type phosphofructose kinase (PFKP) through the Wnt / β-catenin pathway, inhibit the glycolysis of cancer cells and reverse its mediated resistance to cytarabine. Silencing PFKP can effectively inhibit the increase of aerobic glycolysis and epithelial mesenchymal transformation of cancer cells caused by the up-regulation of starvation induced autophagy level [64]. In conclusion, the abnormal activation of autophagy may regulate the reprogramming of glucose metabolism from both positive and negative directions, and thus play a contextual role of cancer inhibition or cancer promotion. The changes in the intricate regulatory relationship between the two may be closely related to the different mechanisms of upstream initiation. The different outcomes induced by different activation pathways have great significance for the understanding and treatment of cancer progression.
In TME, where solid tumors are located, tumor cells whose normal metabolism is restricted can rely on autophagy to survive and reprogram their metabolism to accommodate rapid cell growth and proliferation [10]. Although there have been many research results on the bridging effect of different signaling pathways between cancer cell autophagy and glycolytic reprogramming, it is gradually recognized that the changes of cancer cell autophagy and metabolic reprogramming are closely related to cancer progressive growth, invasion and metastasis, drug resistance formation and even immune escape, etc. However, the mechanism is more inclined to the mutual regulation of autophagy related genes and key glycolysis enzymes, in which the complex initiation and regulatory networks are worth exploring. At present, the interaction between autophagy and Warburg effect in bladder cancer has not been reported, and more in-depth research on it may provide a better understanding of this disease from a new perspective and lay a foundation for exploring the development mechanism of bladder cancer and therapeutic strategies to delay its progression.
None.
Ethical policy
All procedures performed in this study were in accordance with the ethical standards of the institutional and/or national research committee and with the 1964 Helsinki declaration and its later amendments or comparable ethical standards. Informed consent was obtained from all individual participants included in the study. Approval from institutional ethical committee was taken.
Availability of data and materials
All data generated or analysed during this study are included in this publication.
Author contributions
JXP, SP: conception, design of study, literature search and review; CLW: manuscript writting; MJY: approval for the final version of the manuscript and funding supports.
Competing interests
The authors have no competing interest.
Funding
None.
- Siegel RL, Miller KD: Cancer statistics, 2019. CA Cancer J Clin 2019, 69(1): 7-34.
- Martinez Rodriguez RH, Buisan Rueda O, Ibarz L: Bladder cancer: Present and future. Med Clin (Barc) 2017, 149(10): 449-455.
- Ahmadi H, Duddalwar V, Daneshmand S: Diagnosis and Staging of Bladder Cancer. Hematol Oncol Clin North Am 2021, 35(3): 531-541.
- Dobruch J, Oszczudłowski M: Bladder Cancer: Current Challenges and Future Directions. Medicina (Kaunas) 2021, 57(8): 749.
- Yin M, Joshi M, Meijer RP, Glantz M, Holder S, Harvey HA, Kaag M, Fransen van de Putte EE, Horenblas S, Drabick JJ: Neoadjuvant Chemotherapy for Muscle-Invasive Bladder Cancer: A Systematic Review and Two-Step Meta-Analysis. Oncologist 2016, 21(6): 708-715.
- Hu J, Chen J, Ou Z, Chen H, Liu Z, Chen M, Zhang R, Yu A, Cao R, Zhang E et al: Neoadjuvant immunotherapy, chemotherapy, and combination therapy in muscle-invasive bladder cancer: A multi-center real-world retrospective study. Cell Rep Med 2022, 3(11): 100785.
- Jubber I, Ong S, Bukavina L, Black PC, Compérat E, Kamat AM, Kiemeney L, Lawrentschuk N, Lerner SP, Meeks JJ et al: Epidemiology of Bladder Cancer in 2023: A Systematic Review of Risk Factors. Eur Urol 2023, 84(2): 176-190.
- Necchi A, Anichini A, Raggi D, Briganti A, Massa S, Lucianò R, Colecchia M, Giannatempo P, Mortarini R, Bianchi M et al: Pembrolizumab as Neoadjuvant Therapy Before Radical Cystectomy in Patients With Muscle-Invasive Urothelial Bladder Carcinoma (PURE-01): An Open-Label, Single-Arm, Phase II Study. J Clin Oncol 2018, 36(34): 3353-3360.
- David KA, Milowsky MI, Ritchey J, Carroll PR, Nanus DM: Low incidence of perioperative chemotherapy for stage III bladder cancer 1998 to 2003: a report from the National Cancer Data Base. J Urol 2007, 178(2): 451-454.
- Lozy F, Karantza V: Autophagy and cancer cell metabolism. Semin Cell Dev Biol 2012, 23(4): 395-401.
- Yan RL, Chen RH: Autophagy and cancer metabolism-The two-way interplay. IUBMB Life 2022, 74(4): 281-295.
- Chryplewicz A, Scotton J, Tichet M, Zomer A, Shchors K, Joyce JA, Homicsko K, Hanahan D: Cancer cell autophagy, reprogrammed macrophages, and remodeled vasculature in glioblastoma triggers tumor immunity. Cancer Cell 2022, 40(10): 1111-1127.e1119.
- Katheder NS, Khezri R, O'Farrell F, Schultz SW, Jain A, Rahman MM, Schink KO, Theodossiou TA, Johansen T, Juhász G et al: Microenvironmental autophagy promotes tumour growth. Nature 2017, 541(7637): 417-420.
- Hui L, Chen Y: Tumor microenvironment: Sanctuary of the devil. Cancer Lett 2015, 368(1): 7-13.
- Arneth B: Tumor Microenvironment. Medicina (Kaunas) 2019, 56(1).
- Xiao Y, Yu D: Tumor microenvironment as a therapeutic target in cancer. Pharmacol Ther 2021, 221: 107753.
- Netea-Maier RT, Smit JWA, Netea MG: Metabolic changes in tumor cells and tumor-associated macrophages: A mutual relationship. Cancer Lett 2018, 413: 102-109.
- Lyssiotis CA, Kimmelman AC: Metabolic Interactions in the Tumor Microenvironment. Trends Cell Biol 2017, 27(11): 863-875.
- Hanahan D: Hallmarks of Cancer: New Dimensions. Cancer Discov 2022, 12(1): 31-46.
- Hanahan D, Monje M: Cancer hallmarks intersect with neuroscience in the tumor microenvironment. Cancer Cell 2023, 41(3): 573-580.
- Gatenby RA, Gillies RJ: Why do cancers have high aerobic glycolysis? Nat Rev Cancer 2004, 4(11): 891-899.
- Guo D, Tong Y, Jiang X, Meng Y, Jiang H, Du L, Wu Q, Li S, Luo S, Li M et al: Aerobic glycolysis promotes tumor immune evasion by hexokinase2-mediated phosphorylation of IκBα. Cell Metab 2022, 34(9): 1312-1324.e1316.
- Massari F, Ciccarese C, Santoni M, Iacovelli R, Mazzucchelli R, Piva F, Scarpelli M, Berardi R, Tortora G, Lopez-Beltran A et al: Metabolic phenotype of bladder cancer. Cancer Treat Rev 2016, 45: 46-57.
- Warburg O: On the origin of cancer cells. Science 1956, 123(3191): 309-314.
- Koppenol WH, Bounds PL, Dang CV: Otto Warburg's contributions to current concepts of cancer metabolism. Nat Rev Cancer 2011, 11(5): 325-337.
- Thompson CB, Vousden KH: A century of the Warburg effect. Nat Metab 2023, 5(11): 1840-1843.
- Roh JL, Park JY, Kim EH, Jang HJ, Kwon M: Activation of mitochondrial oxidation by PDK2 inhibition reverses cisplatin resistance in head and neck cancer. Cancer Lett 2016, 371(1): 20-29.
- Woolbright BL, Choudhary D, Mikhalyuk A, Trammel C, Shanmugam S, Abbott E, Pilbeam CC, Taylor JA, 3rd: The Role of Pyruvate Dehydrogenase Kinase-4 (PDK4) in Bladder Cancer and Chemoresistance. Mol Cancer Ther 2018, 17(9): 2004-2012.
- Mazurek S, Boschek CB, Hugo F, Eigenbrodt E: Pyruvate kinase type M2 and its role in tumor growth and spreading. Semin Cancer Biol 2005, 15(4): 300-308.
- Mazurek S, Grimm H, Boschek CB, Vaupel P, Eigenbrodt E: Pyruvate kinase type M2: a crossroad in the tumor metabolome. Br J Nutr 2002, 87 Suppl 1: S23-29.
- Mazurek S: Pyruvate kinase type M2: a key regulator of the metabolic budget system in tumor cells. Int J Biochem Cell Biol 2011, 43(7): 969-980.
- Wang X, Zhang F, Wu XR: Inhibition of Pyruvate Kinase M2 Markedly Reduces Chemoresistance of Advanced Bladder Cancer to Cisplatin. Sci Rep 2017, 7: 45983.
- Xi Y, Yuan P, Li T, Zhang M, Liu MF, Li B: hENT1 reverses chemoresistance by regulating glycolysis in pancreatic cancer. Cancer Lett 2020, 479: 112-122.
- Conde VR, Oliveira PF, Nunes AR, Rocha CS, Ramalhosa E, Pereira JA, Alves MG, Silva BM: The progression from a lower to a higher invasive stage of bladder cancer is associated with severe alterations in glucose and pyruvate metabolism. Exp Cell Res 2015, 335(1): 91-98.
- De Duve C, Wattiaux R: Functions of lysosomes. Annu Rev Physiol 1966, 28: 435-492.
- Chandrasekar T, Evans CP: Autophagy and urothelial carcinoma of the bladder: A review. Investig Clin Urol 2016, 57 Suppl 1(Suppl 1): S89-97.
- Klionsky DJ, Petroni G, Amaravadi RK, Baehrecke EH, Ballabio A: Autophagy in major human diseases. EMBO J 2021, 40(19): e108863.
- Yamamoto H, Zhang S: Autophagy genes in biology and disease. Nat Rev Genet 2023, 24(6): 382-400.
- Mandelbaum J, Rollins N, Shah P, Bowman D, Lee JY, Tayber O, Bernard H, LeRoy P, Li P, Koenig E et al: Identification of a lung cancer cell line deficient in atg7-dependent autophagy. Autophagy 2015.
- Zhu J, Tian Z, Li Y, Hua X, Zhang D, Li J, Jin H, Xu J, Chen W, Niu B et al: ATG7 Promotes Bladder Cancer Invasion via Autophagy-Mediated Increased ARHGDIB mRNA Stability. Adv Sci (Weinh) 2019, 6(8): 1801927.
- Wu J, Li W, Ning J, Yu W, Rao T, Cheng F: Long noncoding RNA UCA1 targets miR-582-5p and contributes to the progression and drug resistance of bladder cancer cells through ATG7-mediated autophagy inhibition. Onco Targets Ther 2019, 12: 495-508.
- Ciccarese C, Iacovelli R, Bria E, Mosillo C, Bimbatti D, Fantinel E, Bisogno I, Brunelli M, Tortora G: Second-line therapy for metastatic urothelial carcinoma: Defining the best treatment option among immunotherapy, chemotherapy, and antiangiogenic targeted therapies. A systematic review and meta-analysis. Semin Oncol 2019, 46(1): 65-72.
- Lin JF, Lin YC, Tsai TF, Chen HE, Chou KY, Hwang TI: Cisplatin induces protective autophagy through activation of BECN1 in human bladder cancer cells. Drug Des Devel Ther 2017, 11: 1517-1533.
- Jiménez-Guerrero R, Gasca J, Flores ML: Obatoclax and Paclitaxel Synergistically Induce Apoptosis and Overcome Paclitaxel Resistance in Urothelial Cancer Cells. Cancers (Basel) 2018, 10(12): 490.
- Rakesh R, PriyaDharshini LC, Sakthivel KM, Rasmi RR: Role and regulation of autophagy in cancer. Biochim Biophys Acta Mol Basis Dis 2022, 1868(7): 166400.
- Debnath J, Gammoh N, Ryan KM: Autophagy and autophagy-related pathways in cancer. Nat Rev Mol Cell Biol 2023, 24(8): 560-575.
- Ferro F, Servais S, Besson P, Roger S, Dumas JF, Brisson L: Autophagy and mitophagy in cancer metabolic remodelling. Semin Cell Dev Biol 2020, 98: 129-138.
- Kimmelman AC, White E: Autophagy and Tumor Metabolism. Cell Metab 2017, 25(5): 1037-1043.
- Poillet-Perez L, White E: Role of tumor and host autophagy in cancer metabolism. Genes Dev 2019, 33(11-12): 610-619.
- Currie E, Schulze A, Zechner R, Walther TC, Farese RV, Jr.: Cellular fatty acid metabolism and cancer. Cell Metab 2013, 18(2): 153-161.
- Guo JY, White E: Autophagy, Metabolism, and Cancer. Cold Spring Harb Symp Quant Biol 2016, 81: 73-78.
- Guo JY, White E: Autophagy is required for mitochondrial function, lipid metabolism, growth, and fate of KRAS(G12D)-driven lung tumors. Autophagy 2013, 9(10): 1636-1638.
- Strohecker AM, Guo JY, Karsli-Uzunbas G, Price SM, Chen GJ, Mathew R, McMahon M, White E: Autophagy sustains mitochondrial glutamine metabolism and growth of BrafV600E-driven lung tumors. Cancer Discov 2013, 3(11): 1272-1285.
- Ma J, Feng J, Zhou X: Long non-coding RNA HAGLROS regulates lipid metabolism reprogramming in intrahepatic cholangiocarcinoma via the mTOR signaling pathway. Exp Mol Pathol 2020, 115: 104466.
- Tan VP, Miyamoto S: HK2/hexokinase-II integrates glycolysis and autophagy to confer cellular protection. Autophagy 2015, 11(6): 963-964.
- Tan VP, Miyamoto S: Nutrient-sensing mTORC1: Integration of metabolic and autophagic signals. J Mol Cell Cardiol 2016, 95:31-41.
- Yang X, Cheng Y, Li P, Tao J, Deng X, Zhang X, Gu M, Lu Q, Yin C: A lentiviral sponge for miRNA-21 diminishes aerobic glycolysis in bladder cancer T24 cells via the PTEN/PI3K/AKT/mTOR axis. Tumour Biol 2015, 36(1): 383-391.
- Wang XS, Zhang Z, Wang HC, Cai JL, Xu QW, Li MQ, Chen YC, Qian XP, Lu TJ, Yu LZ et al: Rapid identification of UCA1 as a very sensitive and specific unique marker for human bladder carcinoma. Clin Cancer Res 2006, 12(16): 4851-4858.
- Li Z, Li X, Wu S, Xue M, Chen W: Long non-coding RNA UCA1 promotes glycolysis by upregulating hexokinase 2 through the mTOR-STAT3/microRNA143 pathway. Cancer Sci 2014, 105(8): 951-955.
- Jiao L, Zhang HL, Li DD, Yang KL, Tang J, Li X, Ji J, Yu Y, Wu RY, Ravichandran S et al: Regulation of glycolytic metabolism by autophagy in liver cancer involves selective autophagic degradation of HK2 (hexokinase 2). Autophagy 2018, 14(4): 671-684.
- Pedersen SF, Kramhøft B, Jørgensen NK, Hoffmann EK: Shrinkage-induced activation of the Na+/H+ exchanger in Ehrlich ascites tumor cells: mechanisms involved in the activation and a role for the exchanger in cell volume regulation. J Membr Biol 1996, 149(2): 141-159.
- Zhang G, Zhang Y, Dong D, Wang F, Ma X, Guan F, Sun L: MCT1 regulates aggressive and metabolic phenotypes in bladder cancer. J Cancer 2018, 9(14): 2492-2501.
- Fan Q, Yang L, Zhang X, Ma Y, Li Y, Dong L, Zong Z, Hua X, Su D, Li H et al: Autophagy promotes metastasis and glycolysis by upregulating MCT1 expression and Wnt/β-catenin signaling pathway activation in hepatocellular carcinoma cells. J Exp Clin Cancer Res 2018, 37(1): 9.
- Chen G, Liu H, Zhang Y, Liang J, Zhu Y, Zhang M, Yu D, Wang C, Hou J: Silencing PFKP inhibits starvation-induced autophagy, glycolysis, and epithelial mesenchymal transition in oral squamous cell carcinoma. Exp Cell Res 2018, 370(1): 46-57.
- Wu X, Zhou Z, Xu S, Liao C, Chen X, Li B, Peng J, Li D, Yang L: Extracellular vesicle packaged LMP1-activated fibroblasts promote tumor progression via autophagy and stroma-tumor metabolism coupling. Cancer Lett 2020, 478: 93-106.
- Stenehjem DD, Tran D, Nkrumah MA, Gupta S: PD1/PDL1 inhibitors for the treatment of advanced urothelial bladder cancer. Onco Targets Ther 2018, 11: 5973-5989.
- Lei Q, Wang D, Sun K, Wang L, Zhang Y: Resistance Mechanisms of Anti-PD1/PDL1 Therapy in Solid Tumors. Front Cell Dev Biol 2020, 8: 672.
- Liu J, Chen Z, Li Y, Zhao W, Wu J, Zhang Z: PD-1/PD-L1 Checkpoint Inhibitors in Tumor Immunotherapy. Front Pharmacol 2021, 12: 731798.
Annals of urologic oncology
p-ISSN: 2617-7765, e-ISSN: 2617-7773
Copyright © Ann Urol Oncol. This work is licensed under a Creative Commons Attribution-NonCommercial-No Derivatives 4.0 International (CC BY-NC-ND 4.0) License.